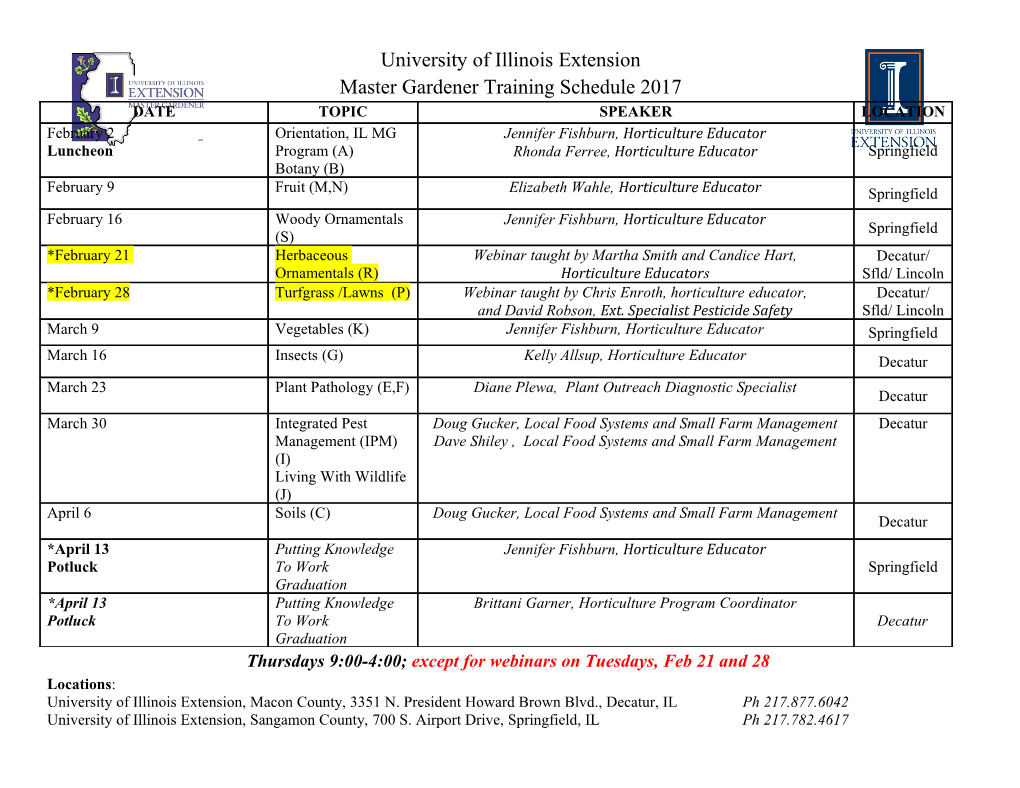
Downloaded from genesdev.cshlp.org on September 23, 2021 - Published by Cold Spring Harbor Laboratory Press REVIEW Not just another hole in the wall: understanding intercellular protein trafficking Kimberly L. Gallagher and Philip N. Benfey1 Department of Biology, Duke University, Durham, North Carolina 27708, USA Development and differentiation of multicellular organ- endomembrane system plays a role in movement to and isms requires cell-to-cell communication. In plants di- through plasmodesmata (for reviews, see Cilia and Jack- rect signaling and exchange of macromolecules between son 2004; Oparka 2004). Intriguing new data regarding cells is possible through plasmodesmata. Recently direct protein movement between animal cells will be re- exchange of membrane-bound vesicles and organelles viewed as well as how these insights might relate to the has been demonstrated between animal cells through mechanisms regulating protein movement in plants. formation of cytoplasmic bridges (tunneling nanotubes) in vitro. Here we review recent developments in cell-to- Holes in walls: plasmodesmata provide cell trafficking of macromolecules in plants and animals. cell-to-cell connectivity Nearly all plant cells are connected to their neighbors by Cell-to-cell communication is essential to coordinate de- plasmodesmata. Plasmodesmata are formed at high den- velopmental events in both animals and plants. Pattern- sity in primary pit fields, areas where the primary walls ing of organs and specification of cell types requires in- of the contacting cells are thin. In function, plasmodes- tercellular signaling to communicate positional informa- mata are similar to gap junctions in that they allow com- tion. The signaling molecules involved in these munication and exchange of ions and metabolites be- developmental events include small molecules, polypep- tween neighboring cells. However, plasmodesmata differ tide ligands, and small RNAs. Surprisingly, in plants, from gap junctions in their ability to allow the passage of transcription factors may also serve as signaling mol- large molecules. In their simplest form, plasmodesmata ecules by trafficking from cell to cell, presumably can be thought of as two concentric cylinders that con- through intercellular channels known as plasmodesmata nect the plasma membranes, cytoplasm, and endoplas- (Lucas et al. 1995; Perbal et al. 1996; Sessions et al. 2000; mic reticulum (ER) of neighboring cells (Fig. 1). The Nakajima et al. 2001; Wada et al. 2002). Recent evidence plasma membrane delimits the outer cylinder of the suggests the possibility that intercellular channels may plasmodesma and connects the neighboring cells. Inter- also exist in animal cells, allowing the transit of macro- nally the ER forms the second cylinder (desmotubule) molecules (Baluska et al. 2004a; Onfelt et al. 2004; Rus- that links the ER in the two adjacent cells. The plasmo- tom et al. 2004). desma thus provides multiple routes for intercellular Determining how proteins move between cells and communication, one through the cytoplasmic space be- what molecules mediate movement is a primary goal in tween the desmotubule and the plasma membrane (often understanding intercellular communication. Since in referred to as the cytoplasmic sleeve or plasmodesmal plants, most protein movement is assumed to occur pore), another via the plasma membrane itself, and a through plasmodesmata, this involves determining how third using the ER (Blackman and Overall 2001; Roberts proteins reach the plasmodesmata and once there, how and Oparka 2003). When first identified, plasmodesmata they move into the neighboring cell. Here we review were largely regarded as fixed structures only allowing recent progress made in understanding the intercellular intercellular movement of molecules small enough to trafficking of the transcription factors LEAFY (LFY) and pass through their cytoplasmic sleeve. It is now clear SHORT-ROOT (SHR). We also discuss work on viral that plasmodesmata are not static. Instead, they are able movement proteins (MPs) that suggests that the plant to modify their size exclusion limit (SEL) on a con- tinuum from open (or dilated), where relatively large [Keywords: Intercellular protein movement; SHORT-ROOT; LEAFY; molecules are able to traffic freely, to closed, where no tunneling nanotubes; protein trafficking; KNOTTED] movement is permitted. 1Corresponding author. In general there are two modes by which molecules E-MAIL [email protected]; FAX (919) 613-8177. Article and publication are at http://www.genesdev.org/cgi/doi/10.1101/ may traffic through plasmodesmata. Cytoplasmically lo- gad.1271005. calized proteins whose molecular weight is below the GENES & DEVELOPMENT 19:189–195 © 2005 by Cold Spring Harbor Laboratory Press ISSN 0890-9369/05; www.genesdev.org 189 Downloaded from genesdev.cshlp.org on September 23, 2021 - Published by Cold Spring Harbor Laboratory Press Gallagher and Benfey More recently, the plant-specific transcription factor LFY has also been shown to move by a nontargeted mechanism (Wu et al. 2003). LFY is required for the tran- sition from vegetative to reproductive development. Both the LFY mRNA and protein are normally expressed in all of the three layers that make up the floral primor- dia (L1, L2, and L3); however, when LFY is expressed in just the L1 layer (epidermis), the protein is able to move into the L2 and L3 layers and to rescue the lfy phenotype. Wu et al. (2003) showed that movement of LFY (47 kDa) in the shoot apical meristem of Arabidopsis is limited in a similar fashion to that of movement of 2XGFP, with Figure 1. Diagram of plasmodesma connecting cells “A” and the extent of protein movement correlating with the “B.” amount of protein present in the cytoplasm. The correlation between the extent of LFY movement plasmodesmatal SEL can move between cells by nontar- and availability in the cytoplasm strongly suggests that geted (or passive) movement. These proteins do not re- LFY movement is nontargeted. To strengthen this argument, quire an interaction with plasmodesmata for movement Wu et al. (2003) made deletions spanning the LFY protein and likely diffuse through the cytoplasmic sleeve. Ex- to determine what effect these mutations would have on amples of nontargeted movement are the cell-to-cell traf- movement, the idea being that if LFY movement were tar- ficking of green fluorescent protein (GFP; Crawford and geted, specific domains within LFY would be required for Zambryski 2001) and the LFY; (Wu et al. 2003) transcrip- interaction with plasmodesmatal associated proteins to tion factor. In contrast, some proteins must interact promote its movement. Mutation or elimination of these with plasmodesmata or associated proteins to increase movement domains should eliminate LFY trafficking. If, the SEL and allow for their own movement. These pro- however, LFY simply diffuses through plasmodesmata, a teins show targeted (or selective) movement. Targeted specific domain would not be required for movement. The movement has been shown for viral MPs, which facili- latter scenario was found; none of the mutations elimi- tate the spread of new viruses between cells, and the nated LFY movement. It is possible that there are redun- KNOTTED1 (KN1; Lucas et al. 1995) and SHR (Galla- dant movement domains in LFY; however, the dependence gher et al. 2004) transcription factors (Zambryski 2004). of LFY movement upon cytoplasmic localization and the lack of any mutations that abolish movement are con- Movement of GFP and LEAFY is nontargeted sistent with the movement of LFY being nontargeted. Proteins that are sufficiently small to move between The conclusion that LFY movement is likely nontar- cells without an increase in the SEL are likely to move geted was somewhat surprising since earlier work by Lee by simple diffusion. This has been shown for cytoplas- et al. (2003) suggested that LFY movement was affected mically localized (free) GFP and LFY (Crawford and Zam- by NON-CELL-AUTONOMOUS PATHWAY PROTEIN bryski 2000; Wu et al. 2003). Free GFP has been shown to 1(NtNCAPP1) from Nicotiana tabacum. NtNCAPP1 move extensively between cells in the Arabidopsis root was identified as a protein that interacted with Cucur- meristem (Imlau et al. 1999; Sena et al. 2004) as well as in bita maxima PHLOEM PROTEIN 16 (CmPP16). Plants the leaf blade. Consistent with movement by diffusion, expressing a dominant negative form of NtNCAPP1 re- multimeric GFP (2XGFP) does not move as extensively as stricted movement of CmPP16 and tobacco mosaic virus monomeric GFP (Crawford and Zambryski 2000, 2001). (TMV) MP but not KN1. Interestingly, these plants had a The subcellular localization of GFP also affects the phenotype similar to overexpression of LFY, suggesting extent of protein movement. In Nicotiana leaf epider- that NtCAPP1 normally interacts with NtLFY and in- mis, addition of a nuclear localization signal to GFP re- hibits its movement. This is in contrast to CmPP16 and duced both the number of cells from which the protein TMV MP whose movement appears to be facilitated by could traffic and the distance traveled. Significantly, NtNCAPP1. It seems possible that NtLFY may move by movement of 2XGFP was nearly blocked by the addition a different mechanism than Arabidopsis LFY or that the of a nuclear localization signal. This was presumably NtNCAPP1 dominant negative indirectly affects diffu- because at ∼54 kDa the dimeric GFP was too large to sion through plasmodesmata. Testing directly whether diffuse freely from the nucleus
Details
-
File Typepdf
-
Upload Time-
-
Content LanguagesEnglish
-
Upload UserAnonymous/Not logged-in
-
File Pages8 Page
-
File Size-