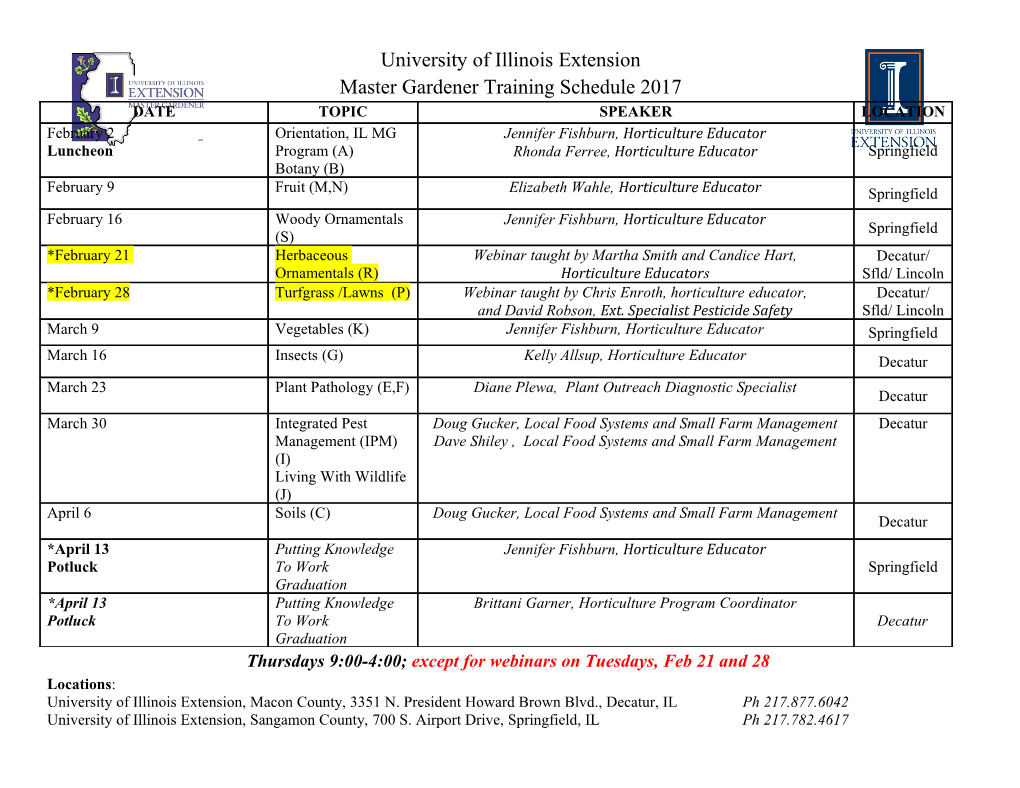
Proc. Natl. Acad. Sci. USA Vol. 89, pp. 75-79, January 1992 Biophysics Chromophore-protein interactions and the function of the photosynthetic reaction center: A molecular dynamics study H. TREUTLEINt, K. SCHULTENt, A. T. BRUNGERt, M. KARPLUS§, J. DEISENHOFER¶, AND H. MICHELII tDepartment of Physics and Beckman Institute, University of Illinois at Urbana-Champaign, Urbana, IL 61801; tHoward Hughes Medical Institute and Department of Molecular Biophysics and Biochemistry, Yale University, New Haven, CT 06511; §Department of Chemistry, Harvard University, Cambridge, MA 02138; $Howard Hughes Medical Institute and Department of Biochemistry, University of Texas Southwestern Medical Center, Dallas, TX 75235-9050; and liMax-Planck-Institut fur Biophysik, D-6000 Frankfurt/Main 71, Federal Republic of Germany Contributed by M. Karplus, December 26, 1990 ABSTRACT The coupling between electron transfer and We demonstrate that a strong coupling between such motions protein structure and dynamics in the photosynthetic reaction and electron transfer exists and that in this respect the RCs center of Rhodopseudomonas viridis is investigated. For this resemble weakly polar solvents with rapid dielectric relax- purpose molecular dynamics simulations of the essential por- ation. The concept that the coupling between thermal motions tions (a segment of5797 atoms) ofthis protein complex have been of charges and dipoles in liquids and proteins plays a role in carried out. Electron transfer in the primary event is modeled electron transfer is not new. The basic ideas go back to the by altering the charge distributions of the chromophores ac- work ofMarcus (3-5) with more recent contributions by many cording to quantum chemical calculations. The simulations show others, as reviewed in ref. 6. In the present study we extend (i) that fluctuations of the protein matrix, which are coupled the earlier work by using molecular dynamics (MD) simula- electrostatically to electron transfer, play an important role in tions to provide a microscopic description for the largely controlling the electron transfer rates and (ii) that the protein phenomenological concepts of the existing models. Such in- matrix stabilizes the separated electron pair state through rapid vestigations have become feasible through the availability of (200 fs) and temperature-independent dielectric relaxation. The high-resolution structures for RCs of the purple bacteria Rp. photosynthetic reaction center resembles a polar liquid in that viridis (7-9) and Rhodobacter sphaeroides (10-12). the internal motions of the whole protein complex, rather than The RC complex of Rp. viridis consists of four different only those of specific side groups, contribute to i and i. The protein subunits, called cytochrome, L, M, and H. In addi- solvent reorganization energy is about 4.5 kcal/mol. The sim- tion, it contains 14 major cofactors: four heme groups are ulations indicate that rather small structural rearrangements covalently attached to the cytochrome subunit; four bacte- and changes in motional amplitudes accompany the primary riochlorophyll b, two bacteriopheophytin b, one menaqui- electron transfer. none 9, one ubiquinone 9, one nonheme iron, and one carotenoid molecule are associated with the subunits L and Photosynthetic reaction centers (RCs) are protein- M (see Fig. 1). Absorption oflight energy by a complex oftwo chromophore complexes in plant and bacterial membranes. bacteriochlorophyll b molecules, the special pair (BCLP and Their function is the conversion of light into a separation of BCMP), leads to a sequence of electron transfer steps within negative and positive charges on different sides of the mem- the RC and to a charge separation across the bacterial inner brane. The RCs carry out their function with a quantum yield membrane (for reviews, see refs. 13 and 14). The first ofthese near unity-i.e., each photon absorbed yields a pair of transfers, from the special pair to one of the bacteriopheo- separated charges. Such a high quantum yield does not phytins (BPL), is very rapid (-3 ps; ref. 15) compared to the necessarily lead to high efficiency since this is determined by back transfer ('10 ns; ref. 13). This step has been observed the quantum yield and the energy content of the separated to require no activation energy, and its rate increases by as charge pair relative to the energy of the photon. In fact, high much as a factor of 2 when the temperature is lowered (16). energy efficiency can be realized for small quantum yields The next electron transfer step, from the bacteriopheophytin when the energy content of the separated charges is in- to one of the quinones (QA), is also much faster ('200 ps; creased. A comparison with solar cells shows that the RCs in refs. 13 and 14) than the back transfer. Recent experiments plants and photosynthetic bacteria are optimized towards have been interpreted as providing evidence that the electron high quantum yields as well as towards high efficiencies- transfer to the bacteriopheophytin may involve a short-lived e.g., the RC of the photosynthetic bacterium Rhodopseudo- intermediate ionization of the bacteriochlorophyll BCLA monas viridis has a quantum yield q > 0.98 (1) and an energy (17); however, the results do not appear to be conclusive. The efficiency 4 = 0.1, whereas optimal solar cells have quantum following considerations apply irrespective of the existence yields of about q = 0.5 and energy efficiencies of about 4 = of such an intermediate. 0.3 (2). An important reason for the emphasis on a high The observed electron transfer rates can be described quantum yield in the biological systems may be the need to phenomenologically in terms of existing electron transfer avoid harmful side reactions, such as the formation of triplet theories (e.g., see refs. 5 and 6). In the present report we intermediates and, possibly, singlet oxygen. demonstrate by means of MD simulations that there is a To achieve a quantum yield near unity, the reactions in- strong coupling between the fluctuating electrostatic inter- volved must form a series of intermediates, and the forward actions ofthe charges localized on the chromophores and the reactions initiated by light excitation must be much faster than protein matrix. The importance of protein fluctuations (i.e., any back reactions. In the present investigation we initiate an low-frequency modes) in electron transfer has been sug- investigation of the relation of the thermal motion of the gested previously (18-21), though specific results concerning components of photosynthetic RCs to electron transfer rates. their role in determining electron transfer rates are not available. We report here the results of MD simulations of a The publication costs of this article were defrayed in part by page charge large (5797 atoms) portion of the RC, including both the payment. This article must therefore be hereby marked "advertisement" in accordance with 18 U.S.C. §1734 solely to indicate this fact. Abbreviations: MD, molecular dynamics; RC, reaction center. 75 Downloaded by guest on September 30, 2021 76 Biophysics: Treudein et al. Proc. Natl. Acad. Sci. USA 89 (1992) functional and the nonfunctional branch (12). A series of simulations were done for the system prior to and after electron transfer to determine the equilibrium structures and fluctuations as well as the relaxation induced by the reaction. Both room temperature and 10 K simulations were per- formed. Preliminary reports on simulations at 300 K have been given by us in refs. 22-24. The present work is a significant N,-~N extension of these studies.** Related work has been per- formed by Warshel and coworkers (29-31).tt Methods The calculations we describe in this article were based on the x-ray structure of the RC of Rp. viridis at 2.3-A resolution and H.M., unpublished work). We FIG. 1. Average structure of chromophores, together with their (J.D., 0. Epp, I. Sinning, abbreviated names in the simulated protein segment after electron used charge distributions of neutral and ionized chro- transfer. Thin black lines denote atom pairs with at least one atom mophores determined by INDO calculations (32), which are in whose positions have changed by <0.4 A, shaded areas correspond good agreement with ESR observations that exhibit an asym- in the same way to atoms whose positions have changed between 0.4 metrical distribution of the positive charge between the two A and 0.7 A, and thick black lines mark atoms with positional monomeric chlorophylls of the special pair. For the excited changes of >0.7 A. state ofthe special pair SP*, we used the ground state charge distribution; the differences between the ground and the tion and buffer region) together with their abbreviated names. excited state of the neutral pair is expected to have a small Light is absorbed by the special pair (BCLP and BCMP). effect. The MD calculations were made with the CHARMM Then an electron is transferred from the special pair to the program (33) and employed the stochastic boundary method bacteriopheophytin BPL, from there via the menaquinone to limit the simulation region (34) to the central part ofthe RC. QA to a ubiquinone QB. Fig. 1 also shows chromophores not A spherical shell of atoms 2.5 A thick around that MD region directly involved in electron transfer, a carotenoid NS1, a was treated by Langevin dynamics to approximate the ther- fourth bacteriochlorophyll BCMA, and a second bacte- mal and frictional effects of the surrounding bath. A total of riopheophytin BPM. The chromophores BCMP, BCMA, and 5726 atoms inside a sphere of29 A around the center of mass BPM are referred to as the "nonfunctional branch" ofthe RC of the porphyrin rings of the special pair and the bacte- chromophores; BCLP, BCLA, BPL, and QA are referred to riopheophytins were included; all chromophores except the as the "functional branch." three more distant heme units of the cytochrome are inside To investigate the characteristics ofthe RC dynamics and to the boundary.
Details
-
File Typepdf
-
Upload Time-
-
Content LanguagesEnglish
-
Upload UserAnonymous/Not logged-in
-
File Pages5 Page
-
File Size-