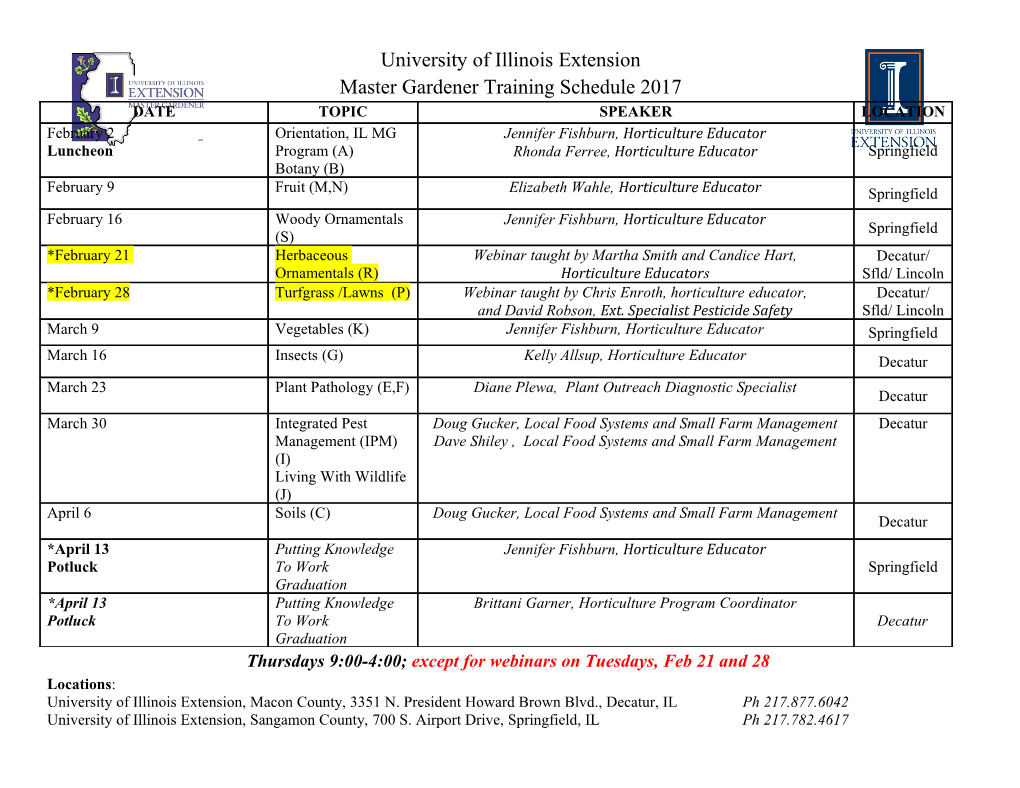
IEEE SENSORS JOURNAL, VOL. 8, NO. 5, MAY 2008 441 Nanofluidics: Systems and Applications Shaurya Prakash, Aigars Piruska, Enid N. Gatimu, Paul W. Bohn, Jonathan V. Sweedler, and Mark A. Shannon Abstract—Nanofluidics presents growing and exciting oppor- Symbol Description tunities for conducting fundamental studies for processes and systems that govern molecular-scale operations in science and Absolute temperature. engineering. In addition, nanofluidics provides a rapidly growing platform for developing new systems and technologies for an Applied electric potential. ever-growing list of applications. This review presents a summary Local electric potential. of the transport phenomena in nanofluidics with a focus on several systems and applications important to problems of public health Current density. and welfare. Special emphasis is afforded to the role of the electric double layer and the molecular-scale interactions that occur Total number of ionic species. within confined nanoscale systems. Inverse Debye length. Index Terms—Microfluidics, nanofluidics, nanomedicine, sur- Debye length. faces, water purification. Diameter of nanochannel/nanopore. Radius of th ionic species. NOMENCLATURE Viscosity. Symbol Description Length of nanochannel/nanopore. Molar flux of th species. Hydraulic diameter. Diffusion coefficient of th species. Pressure drop. Molar concentration of th species. Body force. Velocity of bulk flow. Density. Mobility of th species. Electric field. Valence of th species. Bessel function of the first kind. Faraday’s constant. Zeta potential. Electrical potential. Volumetric flow rate. Universal gas constant. I. INTRODUCTION Manuscript received June 25, 2007; accepted August 12, 2007. This work was supported in part by the National Science Foundation through the HE DIGITAL AGE has seen tremendous advances in sci- Center for Nano-Chemical-Electrical-Mechanical Manufacturing Systems Tence and technology that have propelled human imagina- (Nano-CEMMS) under DMI-0328162, in part by the Science and Technology tion to new heights. The vision of developing massively parallel Center of Advanced Materials for the Purification of Water with Systems (WaterCAMPWS) under Agreement CTS-0120978, in part by the Department microscale systems for automating operations in the chemical of Energy under Grant DE FG02 07ER15851, and in part by the Strategic and biological sciences now seems within reach, and the re- Environmental Research and Development Program (SERDP). The associate search community wants to move to the next level—building editor coordinating the review of this paper and approving it for publication was Dr. Richard Fair. systems to probe, analyze, and use individual molecules to ad- S. Prakash was with the Department of Mechanical Science and Engineering, dress issues in public health and welfare. Toward these goals, University of Illinois at Urbana–Champaign, Urbana, IL 61801 USA. He is now with the Department of Mechanical and Aerospace Engineering, Rutgers, nanofluidics will play an important role as chemistry and bi- The State University of New Jersey, Piscataway, NJ 08854-8058 USA (e-mail: ology occur at molecular length scales. The defining feature [email protected]). of nanofluidics is that transport processes occur within systems A. Piruska, E. N. Gatimu, and P. W. Bohn are with the Department of Chem- ical and Biomolecular Engineering, University of Notre Dame, Notre Dame, IN with critical dimensions on the order of characteristic physical 46556 USA (e-mail: [email protected]; egatimu@nd. du; [email protected]). scaling lengths, typically 1–100 nm. In some nanofluidic appli- J. V. Sweedler is with the Department of Chemistry, University of Illinois at cations, the key length scales are determined by the shielding Urbana–Champaign, Urbana, IL 61801 USA (e-mail: [email protected]). M. A. Shannon is with the Department of Mechanical Science and Engi- properties of aqueous solutions. For example, the Debye length, neering, University of Illinois at Urbana–Champaign, Urbana, IL 61801 USA is nm for deionized (DI) water (limited by (e-mail: [email protected]). the autoprotolysis of water), and is less than 1 nm for concen- Color versions of one or more of the figures in this paper are available online at http://ieeexplore.ieee.org. trated salt solutions. Therefore, in many systems and applica- Digital Object Identifier 10.1109/JSEN.2008.918758 tions the effects of shielding and the electric double layer (EDL) 1530-437X/$25.00 © 2008 IEEE 442 IEEE SENSORS JOURNAL, VOL. 8, NO. 5, MAY 2008 Fig. 1. A schematic description of the length scales and lithographic techniques used in micro and nanosystems. Figure from [1] and [2]. govern transport and reactions in nanofluidic structures. Using II. THEORETICAL BACKGROUND nanoscale systems and functional nanoscale components in mi- In contrast to the recent explosion of interest in nanoflu- croscale devices provide exciting opportunities for building sys- idics, the underlying concepts that govern nanofluidics have tems that can probe or manipulate individual molecules. Fig. 1 been known for some time and are discussed next. The theory shows the length scales important for transport at the micro and assumes that the continuum descriptions of fluid transport are nanoscales. The length scales are presented from three perspec- valid. Once the continuum assumption breaks down, advanced tives: those based on representative devices and applications, the numerical techniques are generally needed for accurate descrip- objects that are important for these devices and applications, and tions of transport phenomena. A discussion of noncontinuum lithographic and fabrication techniques that can be used to fab- models and some key advances can be found in several excel- ricate the devices. lent references [13], [23]–[30]. The advantages of microfluidics, such as limited reagent Most transport phenomena at the nanoscale are driven either consumption, high throughput, and development of disposable by electrokinetic flow or surface mediated transport. Pressure low-cost devices, are well documented [1], [3]–[6]. In addition, driven flows, more common for macroscale systems, are rarely transport physics at the microscale are also well-understood employed for nanoscale systems due to the large driving pres- [1], [7]–[10]. However, the story for nanofluidics is quite sures required, as can be seen from the dependence of pres- different. The walls, due to the large surface-area-to-volume sure drop, , on channel diameter in the Hagen–Poiseuille ratios (as high as m ), dominate behavior giving rise to equation [31] interesting phenomena such as ion permselectivity, ion enrich- ment (or depletion), fast injection of small volumes of reagents, (1) rapid mixing, and pH, charge and concentration gradients stable for long periods of time. New experimental tools such where is the viscosity of the fluid, the volumetric flow rate, as scanning probe microscopy (SPM), confocal microscopy, and the length of the channel. For instance, with water the and single-molecule fluorescence along with the advances in pressure drop across a 100 m long channel 1 nm in diameter for numerical calculations and atomic scale simulation methods only an attoliter ( m ) per second incompressible laminar provide an increased clarity of understanding of the principles flow would need a pressure drop from (1) greater than 3 GPa, on which new applications can be based [8], [10]–[14]. which is impractical for any device. Electrokinetic flows can The purpose of this review is to summarize the transport phe- sustain higher flow rates through nanometer channels without nomena in nanofluidics, with special emphasis on the distinctive excessive pressures. Thus, electrokinetic flows are a preferred role of the EDL and the molecular-scale interactions that occur method for driving fluid and ions through nanochannels. within confined nanoscale systems. The range of devices, how they are fabricated, and the applications differ in many ways A. Electrokinetic Flow from their microscale constructs. Theoretical investigations of A charged surface in contact with a liquid forms an EDL. nanofluidics, including numerical simulations, have focused on The concept of the EDL has been developed over the last cen- nanoscale transport and several excellent references exist [8], tury [16], [17], [32], [33], and several excellent reviews have [10], [12], [13], [15]–[21]. Experimental studies, which con- been presented [18], [19], [34], [35]. A schematic diagram il- stitute the majority of this review, may require special fabrica- lustrating the classic EDL structure is shown in Fig. 2, showing tion approaches, with a recent review highlighting methods of the positions to which different characteristic surface charge re- nanofabrication [22]. lated potentials are referenced within the EDL. Ionic transport PRAKASH et al.: NANOFLUIDICS: SYSTEMS AND APPLICATIONS 443 Fig. 2. A schematic depiction of the classic double-layer structure with the locations where key potentials are measured. Figure based on the work of [16], [23], [25], and [35]. Fig. 3. A simple form of the molecular gate construct. The NCAM acts as is classically described by the Nernst–Planck equation and the a fluidic bridge between microfluidic channels. The NCAM presents exciting velocity profiles are often obtained by using the Navier–Stokes new possibilities in micro-nanoscale systems due to the interesting physics as equations, which are the equations of motion. The potential dis- discussed in this review. (a) shows some SEM images
Details
-
File Typepdf
-
Upload Time-
-
Content LanguagesEnglish
-
Upload UserAnonymous/Not logged-in
-
File Pages10 Page
-
File Size-