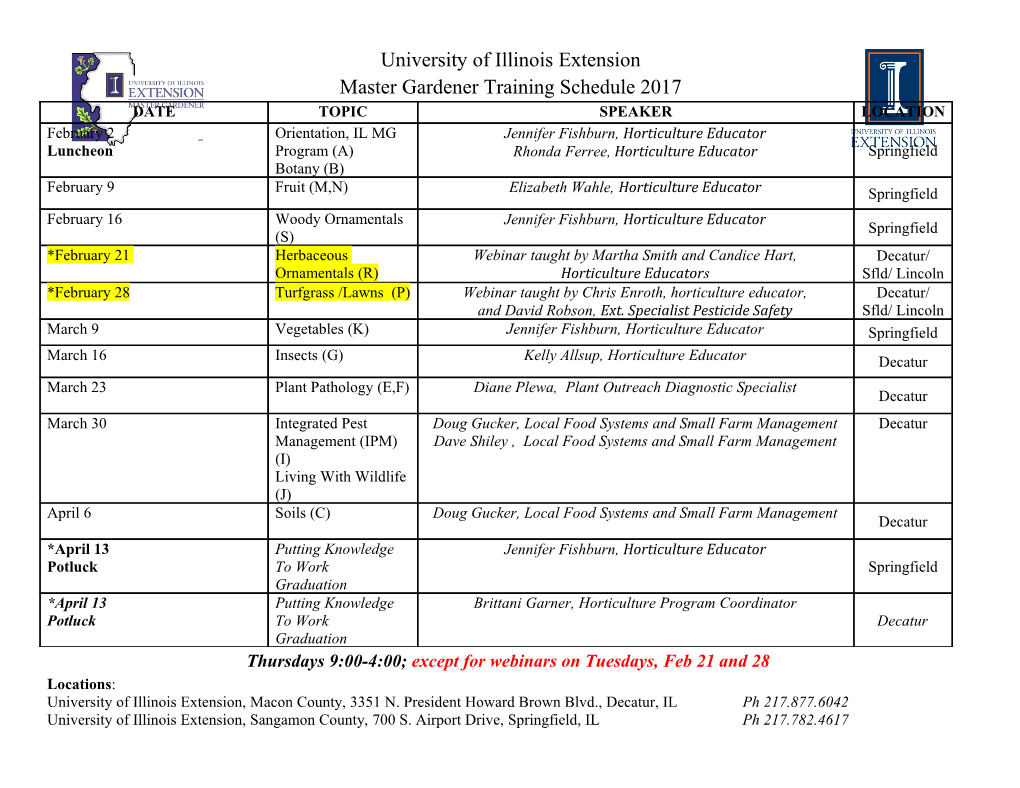
Autotrophy as a predominant mode of carbon fixation in anaerobic methane-oxidizing microbial communities Matthias Y. Kellermanna,1,2,3, Gunter Wegenerb,c,1, Marcus Elverta, Marcos Yukio Yoshinagaa, Yu-Shih Lina, Thomas Hollerc, Xavier Prieto Mollara, Katrin Knittelc, and Kai-Uwe Hinrichsa aOrganic Geochemistry Group, MARUM-Center for Marine Environmental Sciences and Department of Geosciences, University of Bremen, D-28359 Bremen, Germany; bAlfred Wegener Institute for Polar and Marine Research, Research Group for Deep Sea Ecology and Technology, D-27515 Bremerhaven, Germany; and cMax Planck Institute for Marine Microbiology, D-28359 Bremen, Germany Edited by Donald E. Canfield, University of Southern Denmark, Odense M, Denmark, and approved October 5, 2012 (received for review May 24, 2012) The methane-rich, hydrothermally heated sediments of the Guay- autotrophs (20, 21). By contrast, Alperin and Hoehler (22) argued mas Basin are inhabited by thermophilic microorganisms, including on the basis of isotopic considerations that autotrophic metha- anaerobic methane-oxidizing archaea (mainly ANME-1) and sulfate- nogens could equally be responsible for the observations of strongly reducing bacteria (e.g., HotSeep-1 cluster). We studied the microbial 13C-depleted lipids and biomass at numerous cold seep sites. carbon flow in ANME-1/ HotSeep-1 enrichments in stable-isotope– Recently, dual SIP with simultaneous addition of deuterated 13 13 probing experiments with and without methane. The relative incor- water (D2O) and C-labeled inorganic carbon ( CDIC) was in- poration of 13C from either dissolved inorganic carbon or methane troduced as an assay for the quantification of rates of de novo lipid into lipids revealed that methane-oxidizing archaea assimilated pri- synthesis and inorganic carbon assimilation into lipids (23). Fur- 13 marily inorganic carbon. This assimilation is strongly accelerated in thermore, via the relationship of incorporation of CDIC relative the presence of methane. Experiments with simultaneous amend- to D in microbial lipids, lipid biosynthesis by autotrophs and het- ments of both 13C-labeled dissolved inorganic carbon and deuter- erotrophs can be distinguished. To constrain mechanisms and ated water provided further insights into production rates of patterns of carbon flow in microbial communities mediating AOM individual lipids derived from members of the methane-oxidizing 13 we performed a series of SIP experiments with combined CDIC 13 community as well as their carbon sources used for lipid biosynthe- SCIENCES and D2O as well as CH4, using enrichments of AOM-mediating sis. In the presence of methane, all prominent lipids carried a dual microbial communities from the Guaymas Basin (cf. ref. 24). This ENVIRONMENTAL isotopic signal indicative of their origin from primarily autotrophic approach enabled us to differentiate which lipids were produced in microbes. In the absence of methane, archaeal lipid production the presence and absence of methane and to quantify the roles of ceased and bacterial lipid production dropped by 90%; the lipids methane and inorganic carbon as carbon sources for microbes produced by the residual fraction of the metabolically active bacte- involved in AOM. rial community predominantly carried a heterotrophic signal. Collec- tively our results strongly suggest that the studied ANME-1 archaea Results and Discussion oxidize methane but assimilate inorganic carbon and should thus be Activity and Microbial Composition of the Guaymas Basin Enrichments. fi classi ed as methane-oxidizing chemoorganoautotrophs. We incubated replicates of hot seep sediments naturally enriched in moderately thermophilic ANME-1 dominated communities at methanotrophy | biomarker | acetyl-CoA pathway | syntrophy suitable growth conditions (200 kPa CH4,37°C,andartificial seawater medium for sulfate reducers) (25). Samples were amen- 13 13 ethane is an important greenhouse gas and the most abun- ded either with CDIC [resulting C fraction was 9.6% of dissolved Mdant hydrocarbon in marine sediments. Its upward flux to the inorganic carbon (DIC)] and deuterated water (3% deuterium in – 13 13 sediment water interface is strongly reduced by sulfate-dependent total water) or with CH4 (15.9% C of methane carbon) in anaerobic oxidation of methane (AOM) (1, 2). AOM is performed a time series of 10, 17, and 24 d, with and without methane by syntrophic associations of anaerobic methane-oxidizing archaea headspace and in killed controls (Table 1). The incubations with (ANMEs) (3, 4) and their sulfate-reducing bacterial partners methane showed a strong increase of sulfide production in re- Desulfosarcina Desulfobulbus – 13 (SRBs) (mainly relatives of or )(5 8). sponse to stimulation of sulfate reduction. Incubations with CH4 The free energy yield of the AOM net reaction is one of the lowest revealed methane oxidation rates (SI Text, Eq. S1)thatwere – – known for catabolic reactions under environmental conditions (ΔG roughly 85% (3.4 μmol·d 1·g 1) of the sulfate reduction rate – dm ranges from –20 to –40 kJ·mol 1; e.g., refs. 9, 10). Consequently, (Table 1). In the absence of methane, sulfate reduction decreased activity and biomass doubling times determined under optimized by ∼90% (Table 1). Sequencing of 16S rRNA and fluorescence in laboratory conditions range from 2 to 5 mo (11) and growth yields situ hybridization revealed a dominance of aggregate-forming are extremely low, around 1% relative to oxidized methane (11, 12). The biomass of ANMEs and SRBs involved in AOM is usually 13 strongly depleted in C. For instance, at methane seep locations, Author contributions: M.Y.K., G.W., Y.-S.L., and K.-U.H. designed research; M.Y.K., G.W., the δ13Cvaluesofspecific bacterial fatty acids and archaeal ether M.E., M.Y.Y., and T.H. performed research; M.Y.K., M.E., M.Y.Y., X.P.M., and K.K. ana- lipids range from –60 to –100‰ and –70 to –130‰, respectively lyzed data; and M.Y.K., G.W., M.Y.Y., and K.-U.H. wrote the paper. (e.g., refs. 13–17). Such low values have been interpreted as evi- The authors declare no conflict of interest. dence for the incorporation of 13C-depleted methane into biomass This article is a PNAS Direct Submission. (e.g., refs. 3, 4, 18). Data deposition: The nucleotide sequences reported in this paper have been deposited at One way to identify the carbon sources of microbial biomass is to the European Molecular Biology Laboratory, GenBank, and the DNA Data Base of Japan (DDBJ) [accession nos. FR682479–FR682487 and HE817766–HE817767 (archaeal 16S rRNA perform stable–isotope–probing (SIP) experiments (19), followed genes); and FR682618–FR682643 (bacterial 16S rRNA genes)]. by analysis of biomolecules such as membrane lipids (lipid-SIP 1M.Y.K. and G.W. contributed equally to this work. hereafter). Application of lipid-SIP to cold seep sediments and 2Present address: Department of Earth Science and Marine Science Institute, University of microbial mats indicated that archaeal communities dominated by California, Santa Barbara, CA 93106. ANME-2 use both methane-derived carbon and inorganic carbon 3To whom correspondence should be addressed. E-mail: [email protected]. for lipid biosynthesis, whereas their bacterial partners assimilate This article contains supporting information online at www.pnas.org/lookup/suppl/doi:10. only inorganic carbon to produce biomass and are thus complete 1073/pnas.1208795109/-/DCSupplemental. www.pnas.org/cgi/doi/10.1073/pnas.1208795109 PNAS | November 20, 2012 | vol. 109 | no. 47 | 19321–19326 Downloaded by guest on September 26, 2021 Table 1. Overview of conditions in incubation experiments 13 –1 –1 Incubation time Substrate combination δ CDIC, ‰ δDH2O, ‰ H2S production, μmol·d ·gdm Control t0, no label CH4 NA NA NA 13 – t0, + CH4 CH4;D2O; H CO3 NA 244,000 NA 13 13 t0, + CH4 CH4 (∼16,000‰)NANANA 13 – t24, killed CH4;D2O; H CO3 ; ZnCl2 8,500 199,000 0.4 + CH4 t10, + CH4 8,400 196,000 13 – t17, + CH4 CH4;D2O; H CO3 8,200 206,000 6.0 ± 0.4 t24, + CH4 8,000 206,000 13 13 t24, + CH4 CH4 950 NA 4.0 w/o CH4 13 – t24,w/oCH4 D2O; H CO3 8,700 210,000 0.7 13 13 13 Duration of the incubation, substrate combinations (DH2O and CDIC; CCH4), labeling strength (δ CDIC and 13 δ CCH4 of the headspace; δDH2O of the aqueous media), and the sulfide production rate of all analyzed samples incubated at 37 °C are shown. CO2 = 50 kPa; CH4 = 200 kPa; N2 = 200 kPa. + CH4, presence of methane headspace; killed, killed control (ZnCl2, final concentration 2% wt/vol); NA, not analyzed; w/o, without. ANME-1 archaea and partner bacteria from the HotSeep-1 relative to time zero (Δδ) was most pronounced for incubations cluster (Fig. S1 and Table S1). with methane, and Δδ increased fairly linearly with time (Fig. 1 A and B). The extent of label uptake differed between individual Microbial Lipid Distribution and Natural Isotopic Compositions. compounds, with the highest change in ΔδDandΔδ13C found for Concentrations and relative abundances of lipids did not change archaeal phytane and bacterial lipids such as iC16:1ω6,iC18:1ω6, fi signi cantly during the incubations, an observation that is consis- and C18:1ω7 FAs (Table S3). For instance, in the 24-d incubation tent with low growth rates reported for this microbial community with methane, changes in phytane’s δ-values were remarkably (doubling time of 77 d at 37 °C) (24). Ether cleavage of archaeal higher than in biphytanes (Fig. 1 A and B and Table S3). The ΔδD lipids in the total lipid extract released mainly acyclic biphytane, of bacterial C14–C18 FAs was high (∼ +12,000‰) and similar to phytane, and bicyclic biphytane (Fig. S2 A and C and Table S2). that of phytane, whereas Δδ13C was significantly lower in the FAs High relative amounts of biphytanes are derived from glycerol (∼ +380‰;Fig.1A and B and Table S3).
Details
-
File Typepdf
-
Upload Time-
-
Content LanguagesEnglish
-
Upload UserAnonymous/Not logged-in
-
File Pages6 Page
-
File Size-