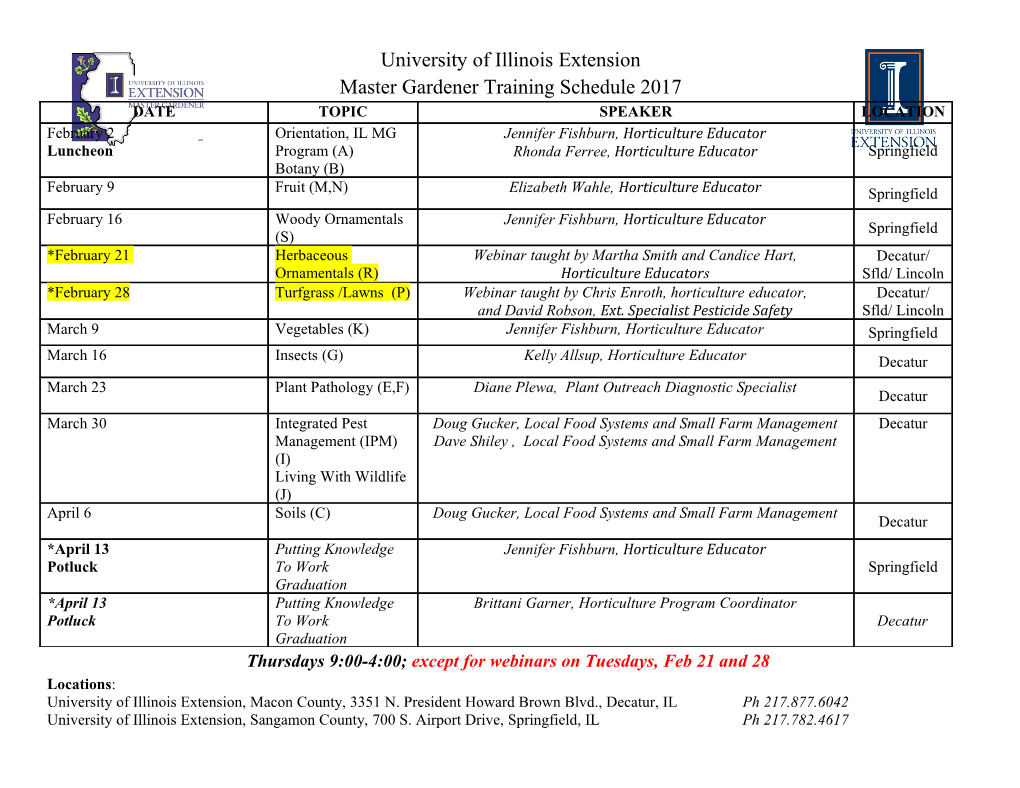
Albert-Ludwigs-Universit¨atFreiburg Fakult¨atf¨urMathematik und Physik Water models and hydrogen bonds Dissertation zur Erlangung des Doktorgrades der Fakult¨atf¨urMathematik und Physik der Albert-Ludwigs-Universit¨atFreiburg im Breisgau Freiburg Institute for Advanced Studies vorgelegt von Roman Shevchuk betreut durch Prof. Dr. Gerhard Stock / Dr. Francesco Rao Freiburg, 2014 Dekan : Prof. Dr. Michal R˚uˇziˇcka Prodekan : Prof. Dr. Andreas Buchleitner Leiter der Arbeit : Prof. Dr. Gerhard Stock Referent : Prof. Dr. Gerhard Stock Koreferent : PD Dr. Thomas Wellens Datum der m¨undlichen Pr¨ufung : 08.05.2014 Contents Introduction 4 1 Molecular simulations 9 1.1 Force fields . 10 1.2 Newtonian dynamics . 11 1.3 Thermostats . 12 1.4 Barostats . 15 1.5 Water models in molecular dynamics . 16 1.6 Simulation details . 20 2 Water phase diagram and water anomalies 22 2.1 Water phase diagram . 22 2.2 Water anomalies . 23 3 Water supercooling and freezing 28 3.1 General perspective . 28 3.2 Test of water freezing . 30 4 Complex network approach for molecular dynamics trajec- tories and hydrogen bond as an order parameter 36 4.1 Complex network as a tool to study molecular simulations . 37 4.2 Hydrogen bond criteria . 43 3 4 CONTENTS 5 Applications 57 5.1 Study of classical water models at ambient pressure . 57 5.2 Effect of polarizability . 67 5.3 Free energy landscape of water . 74 5.4 Proton transfer . 84 Conclusions 97 Bibliography 100 Acknowledgment 122 Introduction For every phenomenon, however complex, someone will eventually come up with a simple and elegant theory. This theory will be wrong. Rotschild's Rule Water is the most important element for all living organisms on Earth. About 80 percents of all living cells consist of water [1]. It plays a role of solvent and thermoregulator, being the environment for the vast majority of all biochemical processes. At the fundamental level, water directly influences several biologically relevant processes including protein folding [2], protein- protein association [2{5] and amyloid aggregation [6]. A single water molecule consists of two hydrogens and an oxygen atom forming a V-shaped molecule with an angle of about 106◦. Because oxygen has a higher electronegativity than hydrogen, the side of the molecule with the oxygen is partially negative and the hydrogen end is partially positive. Consequently, the direction of the dipole moment points from the oxygen towards the center of the hydrogens. This charge difference causes water molecules to be attracted to each other through highly directional hydrogen bonds (the relatively positive areas being attracted to the relatively negative areas) as well as to other polar molecules [7]. One of most interesting properties of water is its polyamorphism. At 5 least 15 crystalline forms of ice are known [8]. For example the number of crystalline modifications of Si or Ge is comparable, but their structural diversity is connected with the transition from semiconductors to metals, on the other hand, the nature of intermolecular interactions in water ice is the same. Water molecules keep their individuality and what changes is the order and structure of the hydrogen bond network [9]. Since there are so many possible crystal structures of water, two questions spontaneously emerge: (i) is there any residual structure in liquid water? (ii) how does water crystallize into ice? To address these questions the concept of network of hydrogen bonds which is continuous in space was proposed by Bernal and Fowler [10]. With all modern experimental and computational techniques there is no doubt that at normal conditions water molecules are connected through three-dimensional network of hydrogen bonds [11, 12]. Many interesting results were obtained by simulations [13{17] and experiments [18,19]. But the problem is that even nowadays none of the experimental methods can track the motion of single water molecules in bulk liquid or explicitly detect all hydrogen bonds in the bulk. This is where computer simulations come into play. The first computer simulation of water was done at the end of the 60s [20,21]. At that time it was possible to simulate a system of a few hundreds of water molecules, where van der Waals interactions were described with a Lennard-Jones potential [22]. With the rise of computational power, the number of simulated molecules increased by several orders of magnitude [23] as well as new refined (and more complex) water models appeared, including molecular flexibility and polarizability [24{27]. In this thesis we will focus on several aspects of molecular dynamics stud- ies of liquid water, particularly the temperature response of some of the most popular water models, including their hydrogen bond network struc- ture. Apart from commonly used thermodynamical measurements here we apply a recently developed complex network framework [16,28]. Within this framework the system is described by a discrete set of a microstates evolv- 6 Introduction ing in time. Microstates represent the nodes of a transition network where a link is placed between two microstates if the system jumped from one to the other one along the molecular dynamics trajectory. Thanks to the net- work analyzing such as cluster structure it is possible to characterize both thermodynamics and kinetics of the system. Combining a complex network framework with more conventional tools like radial distribution function, a detailed description of liquid water is achieved. A short overview of this thesis is presented below: • In Chapter 1 an introduction of the basic principles of molecular dy- namics simulations is provided. The most commonly used approaches for temperature and pressure coupling is described as well as the dif- ference between classical molecular dynamics and Langevin dynamics. • In Chapter 2 the picture of the phase diagram of water is given as well as the description of some of water's properties and so called anomalies. In particular, the water density and thermodynamic anomalies such as presence of the maximum of the density above melting temperature and anomalous increase of viscosity at supercooled region is highlighted. • In Chapter 3 we briefly describe the problems related to supercooled water. The results of the microsecond-long simulation of water in this region are shown, where the correlation between water energy, density and structural order as well as possible scenarios of water freezing were discussed. • In Chapter 4 we give an analysis of the molecular dynamics trajectories via the complex network approach. The detailed description of complex network building for the case of liquid water is provided. In the second section of this chapter the hydrogen bond definitions commonly used in molecular dynamics are analyzed in detail. • In Chapter 5 the applications of above described methods and tools are provided. In particular, the free-energy landscape of water in 220K < 7 T < 340K temperature range is studied via complex network analysis. We present the comparative analysis of seven classical water models as well as the polarizable SWM4-NDP water model. Moreover, the simplified complex network analysis for the case of proton transfer in bulk water is presented. All molecular simulations presented in this thesis (except the ones de- scribed in section 5.4) have been prepared, launched and analyzed by myself. The statistical tools and algorithms used for the analysis have been coded by me in collaboration with Dr. D. Prada-Gracia and in- cluded in a software library called AQUAlab (GPL license, available at raolab.com). 8 Introduction Some results of this thesis were published in the following papers: { R. Shevchuk, D. Prada-Gracia, and F. Rao. Water structure- forming capabilities are temperature shifted for different models. J. Phys. Chem. B., 116(25):7538{7543, 2012. { R. Shevchuk and F. Rao. Note: Microsecond long atomistic sim- ulation of supercooled water. J. Chem. Phys., 137:036101, 2012. { D. Prada-Gracia, R. Shevchuk, P. Hamm, and F. Rao. Towards a microscopic description of the free-energy landscape of water. J. Chem. Phys., 137:144504, 2012. { D. Prada-Gracia*, R. Shevchuk* and F. Rao. The quest for self-consistency in hydrogen bond definitions. J. Chem. Phys., 139:084501, 2013. * authors contributed equally to this work. 9 Chapter 1 Molecular simulations In the recent years along with traditional experiments, computer simulations became a useful tool to elucidate some physical and chemical processes on the molecular level. Here we mainly use classical molecular dynamics sim- ulations, which are a tool that allows to simulate the microscopic system with all-atom resolution using simple Newtonian equations of motion. There are multiple applications of molecular dynamics: they are used for refine- ment of molecular structure from the experiments (crystallography, NMR or electronic microscopy), for the interpretation of the experimental data, for the prediction of functional properties of biological systems and for sampling the regions of phase space which are unreachable in the experiments [29]. First molecular simulations of water were made around forty years ago and were able to calculate the trajectory of few hundreds of atoms for several picoseconds [30]. Since that time the increase of computational power allows simulations to be significantly larger in size and longer in time. Several simu- lations packages such as GROMACS [31], NAMD [32] and LAMMPS [33] al- low to use modern hardware and multiclustering algorithms. Here we briefly describe the basic concepts
Details
-
File Typepdf
-
Upload Time-
-
Content LanguagesEnglish
-
Upload UserAnonymous/Not logged-in
-
File Pages123 Page
-
File Size-