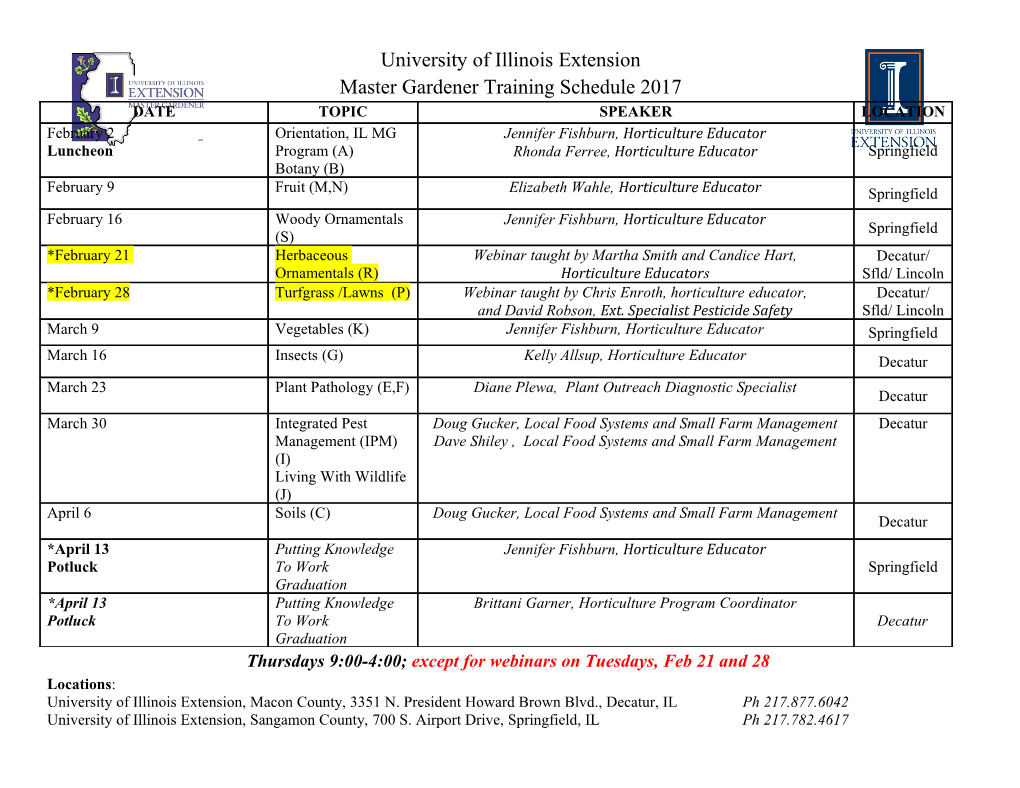
Cyclotides as drug design scaffolds David J Craik* and Junqiao Du Institute for Molecular Bioscience, The University of Queensland, Brisbane, Queensland, 4072, Australia To whom correspondence should be addressed Email: [email protected] Highlights Cyclotides are ultra-stable and tolerant to sequence substitutions in all loops Linear peptide sequences grafted in to a cyclotide scaffold are stabilized Sequences grafted in to a cyclotide scaffold generally maintain biological activity Cyclotides can penetrate cells to deliver bioactive sequences to intracellular targets To date 26 studies of grafted cyclotides have been reported Applications include cancer, pain, cardiovascular disease, obesity and others 1 Abstract Cyclotides are ultra-stable peptides derived from plants. They are around 30 amino acids in size and are characterized by their head-to-tail cyclic backbone and cystine knot. Their exceptional stability and tolerance to sequence substitutions has led to their use as frameworks in drug design. This article describes recent developments in this field, particularly developments over the last two years relating to the grafting of bioactive peptide sequences into the cyclic cystine knot framework of cyclotides to stabilize the sequences. Grafted cyclotides have now been developed that interact with protein or enzyme targets, both extracellular and intracellular, as well as with cell surface receptors and membranes. 2 Introduction Cyclotides [1,2] are disulfide-rich peptides from plants that have a head-to-tail cyclic backbone and cystine knot arrangement of three conserved disulfide bonds, which are linked CysI-CysIV, CysII-CysV and CysIII-CysVI. The combination of the cystine knot motif and cyclic backbone is referred to as a cyclic cystine knot (CCK) and is responsible for the exceptional stability of cyclotides. Their natural function is thought to be plant defence molecules, as they are expressed in a wide range of plant tissues and have activities against insects [3], nematodes [4], and molluscs [5], driven principally by their ability to form pores in membranes [6]. However, the exceptional stability of cyclotides has generated additional interest in their use in protein engineering and drug design applications. Figure 1 shows the sequence and structure of the prototypical cyclotide kalata B1, originally discovered based on its presence in a plant extract used as a uterotonic medicine in the Congo region of Africa in the 1960s. In that application women boiled the above-ground parts of Oldenlandia affinis plants to make a tea which was ingested during labour to accelerate childbirth [7]. This application implies two things: (i) that the peptide survives boiling; and (ii) it survives the digestive tract and is absorbed sufficiently to exert its uterotonic effect. The structure of kalata B1 was first determined in 1995 [8], revealing the CCK motif, which helped to explain the exceptional stability of kalata B1 and its associated favourable biopharmaceutical properties. A wide range of other cyclotides have been discovered since in plants from the Violaceae, Rubiaceae, Cucurbitaceae, Solanaceae and Fabaceae plant families [2] and cyclotide sequences are documented and regularly updated in the publicly accessible database CyBase [9]. It is conservatively estimated that the cyclotide family might comprise more than 50,000 members [10]. Fig 1 here Several other properties, aside from their exceptional stability, have driven pharmaceutical interest in cyclotides. These include their tolerance to substitutions in their backbone loops, their potential for oral bioactivity [11], and most recently, the finding that some cyclotides are able to penetrate cells and 3 thereby potentially interact with intracellular pharmaceutical targets [12,13]. There are currently 380 published papers primarily focused on cyclotides and much of this literature has been extensively reviewed over the last decade. Thus, here we do not attempt to discuss their discovery, natural functions, structural characterisation, or biosynthesis, which have been extensively reviewed elsewhere. (Table 1 provides a list of selected key reviews [14-24] from the last three years that cover these and other background topics). Rather, our focus here is solely on the use of cyclotides as frameworks in drug design [25-40]. These drug design applications have been underpinned by a number of fundamental studies on the synthesis and structural characterisation of cyclotides over the last decade, as schematically illustrated in Figure 1. Furthermore, studies on the cell penetration properties of cyclotides over the last few years have contributed to the possibility of using engineered cyclotides against intracellular pharmaceutical targets. The development of chemical methods for the synthesis of cyclotides has been centrally important to their applications as drug design scaffolds. Specifically, solid phase peptide synthesis and native chemical ligation [41-46] is routinely used to assemble the cyclic backbone of cyclotides, which are well suited to this approach due to their multiple Cys residues. Recombinant methods have also been utilised to make cyclotides [47], as have chemoenzymatic cyclisation approaches [48,49]. These technologies typically are used to make engineered or grafted cyclotides in which one of the native loop sequences is replaced by a loop having a desired bioactivity. Structural studies have been important in the design and validation stages of these applications. Such structural studies of cyclotides have mainly involved NMR methods and there are currently 49 entries for cyclotides or related molecules in the PDB, 42 of which were done with NMR and seven with X-ray crystallography. Table 1 here Over the last decade there have been 52 papers in the literature focusing primarily on drug design applications of cyclotides, including 25 ‘grafting’ papers [11-13,50-71] where the aim is to introduce a 4 desired activity into a cyclotide framework, 11 papers concerning the synthesis/optimisation of the CCK framework [72-82] and 16 reviews on drug design applications of cyclotides [25-40]. This information is summarised in Figure 1, which provides a schematic illustration of how inputs from fundamental studies of cyclotide synthesis, structure and cell penetration, combined with inputs from the literature on the sequences of bioactive peptides have underpinned applications used to design grafted cyclotides to hit specific targets. Figure 2 summarises the grafting concept and highlights that a variety of target receptor types and cellular locations of targets have been explored, ranging from extracellular enzymes to cell surface receptors, membranes, and even intracellular targets, including kinases [13] or protein:protein interactions [12]. In all of these applications just two subfamilies of cyclotide frameworks have been used. Cyclotides are actually divided into three subfamilies, the Möbius, bracelet, and trypsin inhibitor subfamilies. Möbius and bracelet cyclotides are most similar to each other, differing mainly by the presence or absence of a cis-Pro peptide bond in loop 5 of the backbone sequence whereas the trypsin inhibitor cyclotides have quite different sequences. Because bracelet cyclotides are more difficult to correctly fold, almost all grafting studies have focused on Möbius or trypsin inhibitor scaffolds. The fact that bracelet cyclotides cannot practically be used as grafting frameworks is not a major limitation, given the availability of the two other complementary scaffolds, but the development of efficient folding pathways for bracelet cyclotides in future would be valuable to evaluate their potential applications. Recent studies have attempted to unravel the reasons for the difficult folding of bracelet cyclotides by examining chimeric bracelet/Möbius cyclotides [44-46] and a range of other mutated cyclotides. Fig 2 here Table 2 provides an analysis of the grafted cyclotides that have been reported in the literature to date, showing the epitope sizes and grafting locations (i.e. loops) used for the two utilised CCK framework subfamilies (Möbius and trypsin inhibitor). The former subfamily framework tends to be used for 5 extracellular targets and for cases when membrane binding might be advantageous, and the latter for intracellular targets or those involving enzyme inhibition. The table emphasises the vast range of applications of grafted cyclotides, from cancer to metabolic disease, pain and multiple sclerosis. It also emphasises the versatility of the CCK framework and its tolerance to loop substitutions of vastly varying content and size. For example, each and every one of the six backbone loops of the CCK framework has been used to incorporate bioactive epitopes, and the sizes of inserted epitopes have ranged from 1-21 amino acids (Table 2). We note that the grafts involving just single residue substitutions can equivalently be regarded as point mutations, and that such mutations have, in some cases, been used to optimize properties of the framework rather than introducing a target bioactivity per se. For example, single Lys substitutions in kalata B1 were used to increase membrane targeting for enhancing the nematocidal activities of cyclotides [83]. Nevertheless, in Table 2 we classify selected examples of these single-residue substitutions as examples of ‘grafting’ to highlight the generality and limits of the grafting approach. Table 2 here It is clear from Table 2 that most grafting studies have focused on
Details
-
File Typepdf
-
Upload Time-
-
Content LanguagesEnglish
-
Upload UserAnonymous/Not logged-in
-
File Pages27 Page
-
File Size-