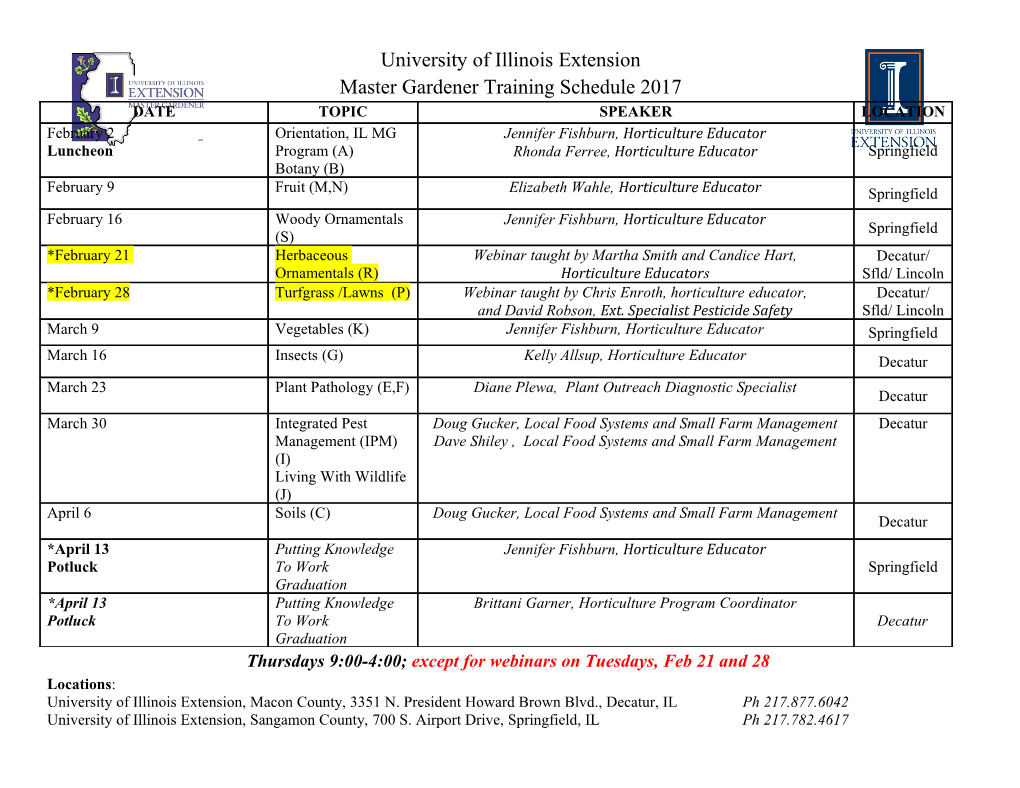
ARTICLES https://doi.org/10.1038/s41558-020-0899-5 Warming drives ecological community changes linked to host-associated microbiome dysbiosis Sasha E. Greenspan 1 ✉ , Gustavo H. Migliorini 2, Mariana L. Lyra 3, Mariana R. Pontes 4,5, Tamilie Carvalho 4,5, Luisa P. Ribeiro 4,5, Diego Moura-Campos 4,5, Célio F. B. Haddad3, Luís Felipe Toledo 4, Gustavo Q. Romero 6 and C. Guilherme Becker1 Anthropogenic climate warming affects many biological systems, ranging in scale from microbiomes to biomes. In many ani- mals, warming-related fitness depression appears more closely linked to changes in ecological community interactions than to direct thermal stress. This biotic community framework is commonly applied to warming studies at the scale of ecosystems but is rarely applied at the scale of microbiomes. Here, we used replicated bromeliad microecosystems to show warming effects on tadpole gut microbiome dysbiosis mediated through biotic community interactions. Warming shifted environmental bacteria and arthropod community composition, with linkages to changes in microbial recruitment that promoted dysbiosis and stunted tadpole growth. Tadpole growth was more strongly associated with cascading effects of warming on gut dysbiosis than with direct warming effects or indirect effects on food resources. These results suggest that assessing warming effects on animal health requires an ecological community perspective on microbiome structure and function. limate warming is a global-scale threat to animal health and optimal richness and composition for pathogen defence and lower fitness1, predominantly through changes in ecological com- indices of dysbiosis, referring to disruption to the normal symbiotic munity interactions2. For instance, warming-mediated shifts host–microbe relationships in the microbiome24. This work sug- C 3 4,5 6,7 in phenology , habitat suitability , food webs and pathogen pres- gests that the functioning of host microbiomes depends on healthy sure8 may interfere with nutrition, reproduction, competitive ability, ecosystem dynamics. Thus, ecosystem disturbances such as climate dispersal and disease defences. Thus, using an ecological commu- warming have the potential to disrupt the community interactions nity framework to investigate effects of climate warming on animal that contribute to microbiome integrity and host fitness. health may reveal important trends that are undetectable outside of We investigated warming effects on amphibian gut bacterial this community context. microbiome dysbiosis mediated through biotic community interac- The host microbiome is essential to many aspects of animal health, tions, a pathway that has received remarkably little attention from including digestion, development, immunity and stress responses9. ecologists. Specifically, we used naturally colonized tank bromeli- Most previous studies investigating warming effects on the micro- ads as model microecosystems (Fig. 1a) to test the hypothesis that biome were not designed to disentangle ecological community fac- warming could influence host fitness through a cascade of effects in tors driving temperature-mediated microbiome shifts10–12, instead which (1) warming influences community assembly of arthropods primarily exploring direct temperature responses of host-associated and/or environmental bacteria, (2) warming-mediated changes bacteria12–16 or indirect responses mediated through host physiol- in the biotic community increase host dysbiosis and (3) dysbiosis ogy17. However, warming effects on biotic community interactions reduces host fitness. To quantify microbiome dysbiosis, we used may be important factors predicting host-associated microbiome the metric known as bacterial dispersion. Dysbiosis is defined as integrity in real-world ecosystems. For instance, warming may alter perturbation of the microbiome that disrupts the symbiotic host– environmental bacterial community composition18,19 or arthropod microbe relationship25. In many cases, dysbiosis is a stochastic pro- community structure, or increase arthropod reproductive rates or cess, leading to increased microbial variability among hosts26. Thus, metabolism, with altered feeding rates leading to changes in the dispersion, a measure of microbial variability, is routinely used as a pool of environmental bacteria20,21 and, subsequently, recruitment measure of dysbiosis26–28. to the microbiome. We placed nursery-raised tank bromeliads in a tropical for- Recent work has begun to resolve how microbiome structure and est in Brazil and used heaters to manipulate water temperature in function are influenced by ecological community interactions19,22–24. bromeliad tanks, with half of the bromeliads exposed to ambient For example, interactions among naturalistic arthropod and bacte- temperatures and the other half warmed up to 6 °C above ambient ria communities within bromeliad tanks had a conditioning effect temperatures, on the basis of predicted climate change scenarios on the environmental bacterial community, enhancing the pool of for 2100 (Fig. 1a,b)29. Bromeliads were then colonized by natural pathogen-inhibiting bacteria and reducing host colonization by communities of arthropods and bacteria for 70 d (hereafter ‘com- transient taxa24. As a result, tadpole microbiome assemblages had munity assembly phase’). We then transferred the bromeliads to a 1Department of Biological Sciences, The University of Alabama, Tuscaloosa, AL, USA. 2Programa de Pós-graduação em Biologia Animal, Universidade Estadual Paulista ‘Júlio de Mesquita Filho’, São José do Rio Preto, Brazil. 3Department of Biodiversity and Aquaculture Center (CAUNESP), Universidade Estadual Paulista, Rio Claro, Brazil. 4Laboratório de História Natural de Anfíbios Brasileiros (LaHNAB), Departamento de Biologia Animal, Universidade Estadual de Campinas, Campinas, Brazil. 5Programa de Pós-Graduação em Ecologia, Instituto de Biologia, Universidade Estadual de Campinas, Campinas, Brazil. 6Departamento de Biologia Animal, Universidade Estadual de Campinas, Campinas, Brazil. ✉e-mail: [email protected] NATURE CLIMATE CHANGE | www.nature.com/natureclimatechange ARTICLES NATURE CLIMATE CHANGE a c ↑ Fast growth rate Slow growth rate ↓ Tadpole gut bacterial dispersion Temperature sensor Tadpole Heater T °C 1 3 Temperature controller Aedes 2 Cool Warm Bacteria Tank bromeliad b n = 5 n = 5 n = 5 n = 5 n = 5 n = 5 Ambient → ambient Ambient → ambient Ambient → ambient Ambient → +4 °C +4 °C → ambient +4 °C → +4 °C n = 5 n = 5 n = 5 n = 5 n = 5 n = 5 Ambient → +2 °C +2 °C → ambient +2 °C → +2 °C Ambient → +6 °C +6 °C → ambient +6 °C → +6 °C Community assembly phase → laboratory phase Fig. 1 | Bromeliad set-up and main experimental findings. a, Bromeliad heating system and focal components of each bromeliad microecosystem. b, Temperature treatments for 60 bromeliads distributed in five replicate spatial blocks. Each bromeliad is divided in half, representing the temperature treatment during the community assembly phase (left) and laboratory phase (right) of the experiment. c, Conceptual diagram illustrating three biotic pathways through which warming influenced tadpole gut bacterial dispersion and growth, with lower bacterial dispersion depicted as an ordered colour spectrum and higher bacterial dispersion depicted as scrambled colours. laboratory (hereafter ‘laboratory phase’) to reduce daily tempera- Warming shifted community assembly of environmental ture fluctuations, added omnivorous but primarily algae-eating bacteria larvae (tadpoles) of the bromeliad-specialist frog species Ololygon Warming-mediated shifts in unweighted UniFrac community com- perpusilla (Lutz & Lutz, 1939) as model hosts30 and switched half position of environmental bacteria were associated with higher of the bromeliads to the opposite temperature treatment (ambient Jaccard dispersion of tadpole gut bacteria (Figs. 1c (red pathway no. to warmed or vice versa; Fig. 1b). Our fully crossed experimen- 1) and 2, Supplementary Fig. 1 and Supplementary Tables 1a,b, 2a,b, tal design allowed us to disentangle (1) indirect effects of warm- 3a,b and 4). We detected 313 amplicon sequence variants (ASVs) ing on host dysbiosis and fitness, specifically through natural, that were unique to the environmental bacterial community (domi- temperature-mediated community assembly, from (2) direct effects nated by order Burkholderiales within phylum Proteobacteria), 277 of warming on host dysbiosis, algal food production and growth. ASVs that were unique to tadpole gut samples (dominated by order Piecewise structural equation models (SEMs) revealed cascad- Clostridiales within phylum Fermicutes) and 388 ASVs that were ing effects of warming on tadpole microbiome dysbiosis through shared between these two groups (dominated by diverse orders several distinct, non-mutually exclusive biotic pathways and linear within phylum Proteobacteria). Seven ASVs were detected in at least mixed-effects models (LME) supported the trends in our SEMs. By 90% of tadpole gut samples, including three ASVs from the animal testing for links among temperature, biotic community structure, gut-associated families Ruminococcaceae and Veillonellaceae (order dysbiosis and host fitness, our study sheds new light on the impacts Clostridiales), three ASVs from the environment-associated fami- of global change on animal health and ecosystem integrity. lies Methylocystaceae (order Rhizobiales) and Microbacteriaceae NATURE CLIMATE CHANGE | www.nature.com/natureclimatechange NATURE CLIMATE CHANGE ARTICLES a b
Details
-
File Typepdf
-
Upload Time-
-
Content LanguagesEnglish
-
Upload UserAnonymous/Not logged-in
-
File Pages8 Page
-
File Size-