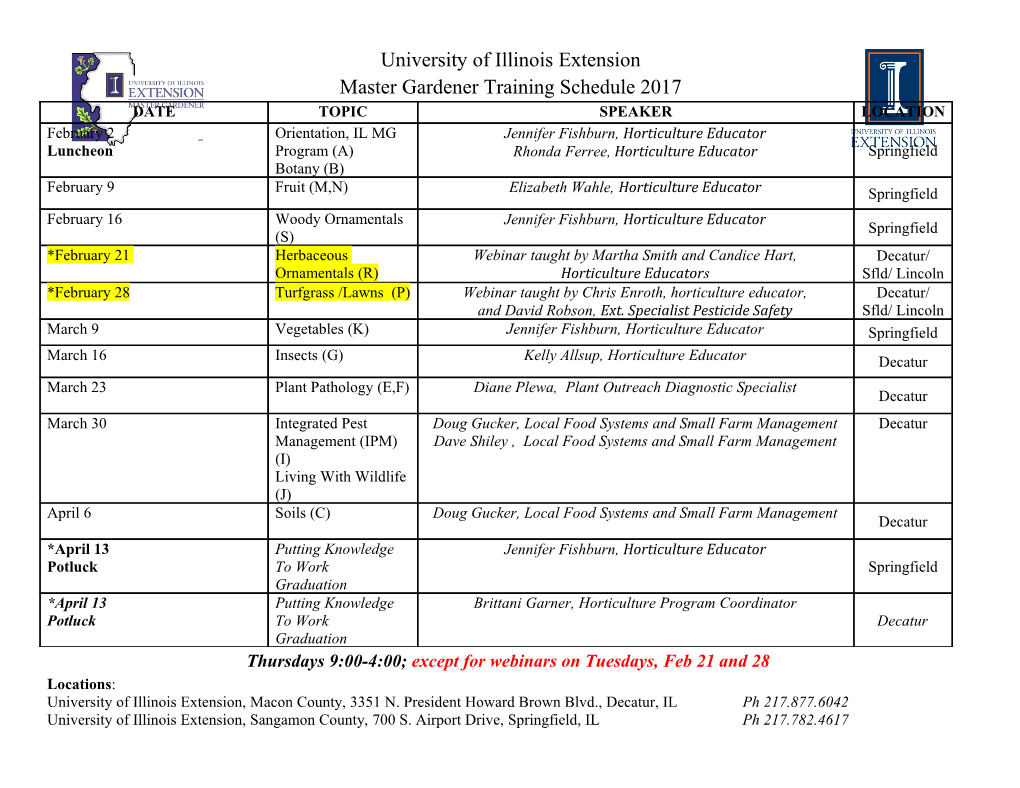
© 2019 ELENA ALEKSANDROVNA SILANTYEVA ALL RIGHTS RESERVED FUNCTIONALIZED NANOFIBER SUBSTRATES FOR NERVE REGENERATION A Dissertation Presented to The Graduate Faculty of The University of Akron In Partial Fulfillment of the Requirements for the Degree Doctor of Philosophy Elena A. Silantyeva May 2019 FUNCTIONALIZED NANOFIBER SUBSTRATES FOR NERVE REGENERATION Elena Aleksandrovna Silantyeva Dissertation Approved: Accepted: _______________________________ _____________________________ Advisor Department Chair Dr. Matthew L. Becker Dr. Tianbo Liu _______________________________ _____________________________ Committee Member Dean of College Dr. Li Jia Dr. Ali Dhinojwala _______________________________ _____________________________ Committee Member Dean of the Graduate School Dr. Chrys Wesdemiotis Dr. Chand Midha _______________________________ _____________________________ Committee Member Date Dr. Darrell Reneker _______________________________ Committee Member Dr. Rebecca Willits iii ABSTRACT Peripheral nerve injuries are a significant clinical challenge due to the limited capacity of nerve tissue to regenerate.1 Recently, research has focused its attention on the polymer nanofiber substrates since they mimic ECM topography and can be functionalized with bioactive molecules to overcome the limitations of both naturally occurring proteins and synthetic polymers used in nerve tissue regeneration applications. In the present work, we have developed poly(ԑ-caprolactone) (PCL) nanofiber substrates, modified with peptides to control the cellular activity. High molecular mass PCLs with a 4- dibenzocyclooctynol initiator was synthesized via ring-opening polymerization using magnesium 2,6-di-tert-butyl-4-methylphenoxide catalyst. The efficient chemical methods of nanofiber surface functionalization, such as strain-promoted azide-alkyne cycloaddition (SPAAC), developed by the Becker Lab,2 and thiol-ene reaction were utilized to introduce biomolecules. The dissertation is separated into four parts and demonstrates the combination of topographical cues and surface-tethered bioactive factors in effort to understand the optimal substrates for nerve regeneration or repair. We utilized the two most promising clinical approaches from our point of view for nerve tissue regeneration. The first approach is the differentiation of stem cells in vitro, described in the first two parts. Substrates that facilitate the differentiation and support long term culture of mature neural cells would advance the field of regenerative medicine. First, we demonstrated that YIGSR-functionalized aligned nanofibers can be potentially used for stem cells neural differentiation in vitro. The commitment and maturity of mESCs on these iv substrates were characterized using gene and protein expression. Then we compared influence of nanofiber topography and bioactive factors on neural differentiation of mESCs. These results highlight the influence of RGD binding versus topography in differentiation through high levels of glial fibrillary acidic protein expression. The second approach is improving Schwann cell migration and is described in the last two parts. Improved Schwann cell response on functionalized nanofibers in vitro was demonstrated in our lab.3 In the present study, we examined RGD-functionalized fibers in vivo, on the rat sciatic nerve model and demonstrated enhancement of Schwann cell infiltration and functional outcomes. To further improve Schwann cell migration, we created nanofiber substrates with gradients of bioactive factors along the aligned fiber direction. All the parts describe efforts to increase control over cellular activity (stem cell neural differentiation or Schwann cell migration) through combination of nanofiber topography and chemically tethered bioactive peptides. Each of these studies help to get an insight into optimal tissue engineering constructs for nerve regeneration. v DEDICATION I would like to dedicate this work to my sister Nataliya Silantyeva and my parents Nina and Aleksandr, for their love and support. vi ACKNOWLEDGEMENTS During my PhD study I was lucky to meet nice people who improved me a scientist as well as a person. This work could not have been completed without the contribution of many individuals. First and foremost, I would like to express my gratitude to my advisor, Dr. Matthew L. Becker, who inspires me to be creative and independent thinker, for giving me so many opportunities and always being supportive. I would like to thank Dr. Willits and her students (Diana Philip, Wafaa Nasir, Jacqueline Carpenter, Elham Malekzadeh, Galina Pylypiv and McKay Cavanaugh) for teaching me how to work in a collaborative environment, for all the advices and help with biological analysis and interpretation. The work of this dissertation would not be possible without the discussions and collaboration with Dr. Willits. I would like to thank Dr. Darrell Reneker for helping me with electrospinning and teaching me how to think ‘outside of the box’. I also want to thank my committee members Dr. Li Jia, Dr. Chrys Wesdemiotis for their advice and constructive feedback to my research. I would like to thank Melissa Bowman, Jacqueline Clark and Marjorie Parrish for their help. I would also like to expand a big thank you to all Becker group members for collaborative and friendly work environment. A special thank you to Saranshu Singla, Fadi Haso and Selemon Bekele for helping me in studying for classes and cumulative exams. Ed Laughlin, Jack Gillespie and Bojie Wang for helping me and suggesting ideas. Finally, I want to thank my family for the constant support. vii LIST OF ABBREVIATIONS ACS American Chemical Society ASCs Adipose-derived stem cells bFGF Basic fibroblast growth factor BMSCs Bone marrow stromal cells ε-CL ε-Caprolactone CuAAC Copper(I)-catalyzed azide-alkyne cycloaddition DCM Dichloromethane DIBO 4-Dibenzocyclooctynol DMF N,N-Dimethylformamide DOSY Diffusion ordered spectroscopy DP Degree of polymerization Đm Molecular mass distribution ECM Extracellular matrix EPT Extensor postural thrust ESI Electrospray ionization GDNF Glial cell-derived neurotrophic factor HFIP 1,1,1,3,3,3-hexafluoro-2-propanol HPLC High performance liquid chromatography mESCs Mouse embryonic stem cells Mn Number average molecular mass Mw Weight average molecular mass viii MD% Percentage of motor deficit %MPE Percent maximal possible effect NGC Nerve guidance conduits NGF Nerve growth factor NIH National Institutes of Health NMR Nuclear magnetic resonance PBS Phosphate-buffered saline PCL Poly(ε-caprolactone) PLGA Poly(lactic-co-glycolic acid) PLLA Poly(L-lactide) PNI Peripheral nerve injury qPCR Quantitative polymerase chain reaction RGMW Relative gastrocnemius muscle weight SC Schwann cell SEC Size exclusion chromatography SEM Scanning electron microscopy SFI Sciatic functional index SPAAC Strain-promoted azide-alkyne cycloaddition THF Tetrahydrofuran TLC Thin-layered chromatographic analysis ix TABLE OF CONTENTS LIST OF TABLES ......................................................................................................... xiii LIST OF FIGURES ....................................................................................................... xiv CHAPTER I. INTRODUCTION ......................................................................................................... 1 1.1. Peripheral nerve injury and its treatment .......................................................... 1 1.2. Stem cell differentiation for nerve injury treatment ............................................ 4 1.3. Electrospun scaffolds for cell culture in nerve regeneration .............................. 4 1.4. Touch-spinning ................................................................................................. 6 1.5. Strain-Promoted Azide-Alkyne Cycloaddition (SPAAC) .................................... 6 II. MATERIALS AND INSTRUMENTATION .................................................................... 9 2.1. Materials .......................................................................................................... 9 2.2. Instrumentation ...............................................................................................10 III. ACCELERATED NEURAL DIFFERENTIATION OF MOUSE EMBRYONIC STEM CELLS ON ALIGNED GYIGSR-FUNCTIONALIZED NANOFIBERS ..............................13 3.1. Abstract ...........................................................................................................13 3.2. Introduction .....................................................................................................14 3.3. Experimental ...................................................................................................16 3.4. Results ............................................................................................................26 3.5. Discussion .......................................................................................................36 3.6. Conclusions .....................................................................................................42 3.7. Acknowledgement ...........................................................................................43 x IV. RGD-FUNCTIONALIZED NANOFIBERS INCREASE EARLY GFAP EXPRESSION DURING NEURAL DIFFERENTIATION OF MOUSE EMBRYONIC STEM CELLS .......44 4.1. Abstract ...........................................................................................................44 4.2. Introduction .....................................................................................................45
Details
-
File Typepdf
-
Upload Time-
-
Content LanguagesEnglish
-
Upload UserAnonymous/Not logged-in
-
File Pages192 Page
-
File Size-