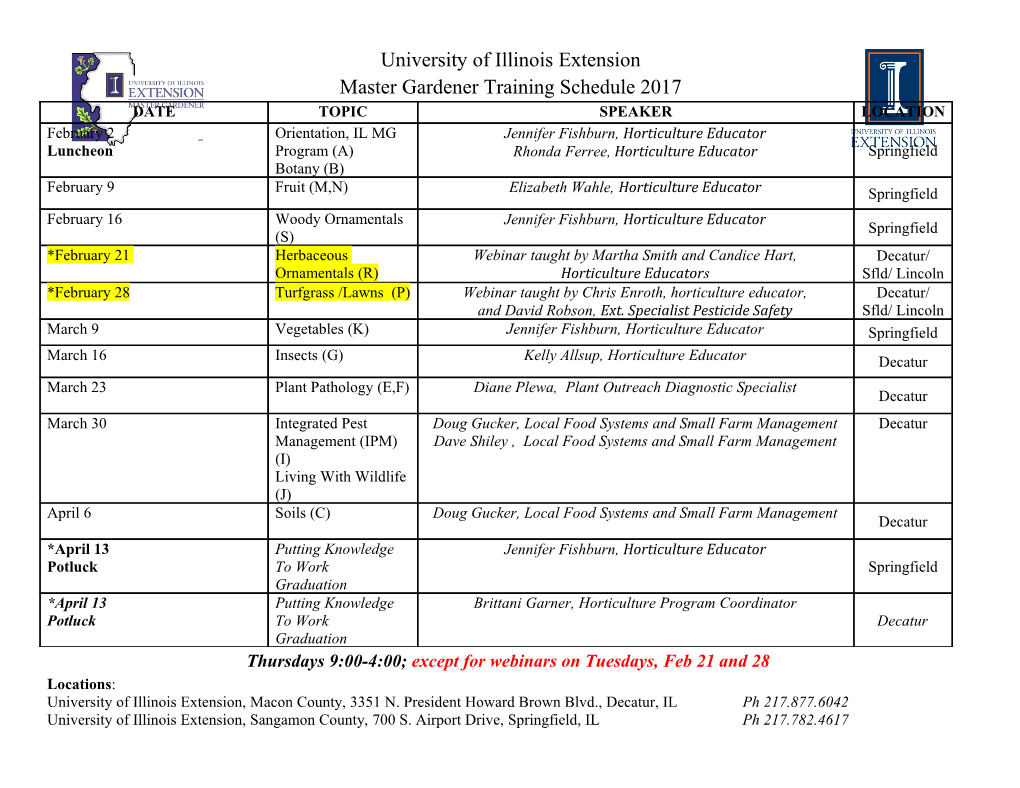
Chemical Geology 532 (2020) 119346 Contents lists available at ScienceDirect Chemical Geology journal homepage: www.elsevier.com/locate/chemgeo Two distinct mantle sources beneath the Garibaldi Volcanic Belt: Insight from olivine-hosted melt inclusions T Swetha Venugopala,b,*, Séverine Mouneb,c,d, Glyn Williams-Jonesa, Timothy Druittb, Nathalie Vigourouxa,e, Alexander Wilsonf, James K. Russellf a Centre for Natural Hazards Research, Department of Earth Sciences, Simon Fraser University, BC, V5A 1S6, Canada b Laboratoire Magmas et Volcans, Université Clermont Auvergne, Campus Universitaire des Cézeaux, 63178, Aubiere Cedex, France c Observatoire Volcanologique et Sismologique de Guadeloupe (OVSG), Le Houëlmont, 97113, Gourbeyre, Guadeloupe d Université de Paris, Institut de Physique du Globe de Paris, CNRS, F-75005, Paris, France e Department of Earth and Environmental Sciences, Douglas College, BC, V3M 5Z5, Canada f Department of Earth, Ocean and Atmospheric Sciences, The University of British Columbia, BC, V6T 1Z4, Canada ARTICLE INFO ABSTRACT Editor: Donald Dingwell The nature of the magmatic source beneath the Garibaldi Volcanic Belt (GVB) in NW Washington (USA) and SW fi Keywords: British Columbia (Canada) has been debated both due to its classi cation as the northern equivalent of the High Melt inclusions Cascades and the alkaline nature of northern basalts. Whole rock studies have shown that the GVB does not share Garibaldi Volcanic Belt the same magmatic source as the High Cascades (Mullen and Weis, 2013, 2015). Nonetheless, the presence of Cascades alkaline basalts in this arc raises questions about the exact source of mantle enrichment and whether it is related Trace elements to the young age of the downgoing Juan de Fuca Plate (< 10 Ma) or the presence of a slab tear at the northern Two mantle sources end of the arc. To gain insight into the source that underlies the GVB, we sampled olivine-hosted melt inclusions from each volcanic centre along the arc. Major, volatile and trace element data reveal a northward compositional trend from arc-typical calc-alkaline magma in the south to OIB-like melts in the north near the slab tear. Fur- thermore, contributions from the subducting slab are relatively high beneath the southern end of the arc (Cl/ Nb > 80) but rapidly decreases to the north (Cl/Nb < 50). Finally, the significant differences in Zr/Nb from south to north (80 and 9, respectively) suggest two distinct mantle sources since one source cannot produce melts with such different ratios. As such, we propose the GVB should be segmented into the Northern and Southern groups, each having its own mantle source. Based on the geographic proximity, the enriched nature of the Northern group melt inclusions is likely controlled by the slab tear at the northern termination of the subducting Juan de Fuca Plate. Melt modelling results show that 3–7 % partial melting of the primitive mantle with a garnet lherzolite residue can reproduce the composition of the Northern group. Melt inclusions from the Southern group, on the other hand, imply a depleted MORB mantle that has been modified by fluids derived from the downgoing slab. Variability within the Southern group itself reflects the amount of hydrous fluids supplied beneath each centre and is correlated with slab age and subsequent degree of dehydration. This study addresses the compositional diversity along the arc and provides evidence that the age of the downgoing plate and the presence of a slab tear exert a strong compositional control over eruptive products within one arc. 1. Introduction Stern, 2018). The presence of faults and fractures can facilitate the rise of more primitive melt by acting as favourable pathways (e.g., Connor The main compositional controls of eruptive products in subduction and Conway, 2000; Gaffney et al., 2007; Venugopal et al., 2016) zone settings are arguably the age and tectonic context of the down- whereas slab tears and slab windows can contribute melts derived from going slab (Cooper et al., 2012; Saginor et al., 2013). Dictated by the the deeper mantle that do not carry the arc signature (e.g., Gorring and age, factors such as slab temperature, subduction angle and the amount Kay, 2001). of slab-derived fluids can cause significant variations in the composi- Modelling of slab thermal structure has shown that older slabs, such tion of the wedge-generated melt (Saginor et al., 2013; Weller and as the Pacific Plate beneath the Aleutian Islands, generally have steeper ⁎ Corresponding author at: Centre for Natural Hazards Research, Department of Earth Sciences, Simon Fraser University, BC, V5A 1S6, Canada. E-mail address: [email protected] (S. Venugopal). https://doi.org/10.1016/j.chemgeo.2019.119346 Received 5 February 2019; Received in revised form 11 June 2019; Accepted 19 October 2019 Available online 21 October 2019 0009-2541/ © 2019 Elsevier B.V. All rights reserved. S. Venugopal, et al. Chemical Geology 532 (2020) 119346 Fig. 1. Regional map of the Cascade Volcanic Arc along western North America. This arc is separated into the High Cascades from northern California to northern Washington and the Garibaldi Volcanic Belt (GVB) from northwest Washington to southwest Canada. Beneath the GVB, the subducting Juan de Fuca Plate youngs in age from 10 Ma below Glacier Peak to 5 Ma beneath Mount Meager where it terminates against the Nootka Fault beneath Salal Glacier and Bridge River. subduction angles, have lower slab temperatures at a given depth, and lower dip angle (Candie et al., 1989; Wilson, 2002). This difference of supply a higher flux of fluids to the sub-arc mantle (Syracuse et al., dip produces a gap or slab tear between the two plates (Thorkelson 2010; Ohtani et al., 2004; Stern, 2002; Ulmer, 2001). Younger slabs, on et al., 2011). the other hand, achieve higher temperatures at a given depth and lower The relatively older Juan de Fuca Plate beneath the southern slab dips (Syracuse et al., 2010). Though poorly investigated, the re- Cascades is capable of supplying more subduction-derived fluids into lease of fluids (due to dehydration reactions) from young slabs has been the mantle wedge, thereby initiating partial melting and producing arc- shown to be at shallower depths than in older slab settings (Ruscitto typical calc-alkaline magma (Green and Sinha, 2005). In terms of the et al., 2010; Walowski et al., 2016). This suggests that ascending pri- GVB, however, little is known of primitive melt compositions and vo- mary derived from young, dehydrated slabs should melts should be latile contents. What is known is the coeval presence of calc-alkaline depleted in volatiles and slab-derived elements. rocks in the southern GVB and alkaline rocks in the northern GVB An excellent case study for studying the effect of slab age and tec- (Green, 2006; Mullen and Weis, 2013, 2015). The GVB therefore pro- tonic framework on the resulting eruptive compositions is the Juan de vides an opportunity to examine both the effects of slab age and slab Fuca Plate, which is considered to be the young and hot end member in tearing on the magmatic source composition and volatile contents global subduction zone systems (Syracuse et al., 2010). The north- within a single subduction zone system. easterly subduction of the Juan de Fuca Plate beneath the North Here we present olivine-hosted melt inclusion data for the volcanic American Plate produces the Cascades Volcanic Arc along the western centres within the Canadian segment of the GVB alongside melt inclu- margin of North America (Fig. 1; Moore and DeBari, 2012). This arc is sion data from Glacier Peak and Mount Baker compiled by Shaw subdivided into the High Cascades in the south and the Garibaldi Vol- (2011). Major element, volatile and trace element contents of the melt canic Belt (GVB) to the north (Fig. 1). The age of the Juan de Fuca Plate inclusions are used to decipher the dynamics of the GVB sub-arc mantle at the trench decreases northward from 10 Ma beneath the northern and to better understand the effect of slab age and slab tear on the end of the High Cascades to approximately 5 Ma beneath the GVB compositions of mantle melts. where it terminates against the Explorer Plate (Fig. 1), which has a 2 S. Venugopal, et al. Chemical Geology 532 (2020) 119346 2. Geological context of the GVB entrapped with the silicate melt. These melt inclusions also contained daughter crystals, which typically form due to volatile loss, slow ascent The subdivision of the Cascades Arc is based on the change in strike rates and/or slow cooling following eruption (Schiano, 2003; Wallace, of the arc axis and a bend in the alignment of volcanic centres; the 2005; Supplementary Figure S1). As such, these inclusions are not re- change in strike is evident between Mount Rainier and Glacier Peak presentative of the original magma. To reverse the daughter crystal- (Fig. 1; Mullen et al., 2017). The High Cascades, which strikes north- lisation process and retrieve the original melt compositions, inclusions south, stretches between northern California and northern Washington. from Bridge River, Salal Glacier, Mount Cayley and Garibaldi Lake were The GVB, on the other hand, is a Quaternary-aged glaciovolcanic arc reheated at the Laboratoire Magmas et Volcans (LMV) in Clermont- extending between northern Washington and southwestern British Co- Ferrand, France. Re-heating experiments for each inclusion were lumbia. This belt strikes northwest-southeast and terminates in the 15 min in duration to reduce volatile loss (Chen et al., 2011; Portnyagin north against the Nootka Fault (Fig. 1). Volcanism within the GVB has et al., 2008; Gaetani et al., 2012; Bucholz et al., 2013) and stopped resulted in more than 2300 distinct vents and 22 major volcanic when the final daughter crystal disappeared (see Supplementary structures, including Glacier Peak, Mount Baker and the volcanic fields Methods).
Details
-
File Typepdf
-
Upload Time-
-
Content LanguagesEnglish
-
Upload UserAnonymous/Not logged-in
-
File Pages12 Page
-
File Size-