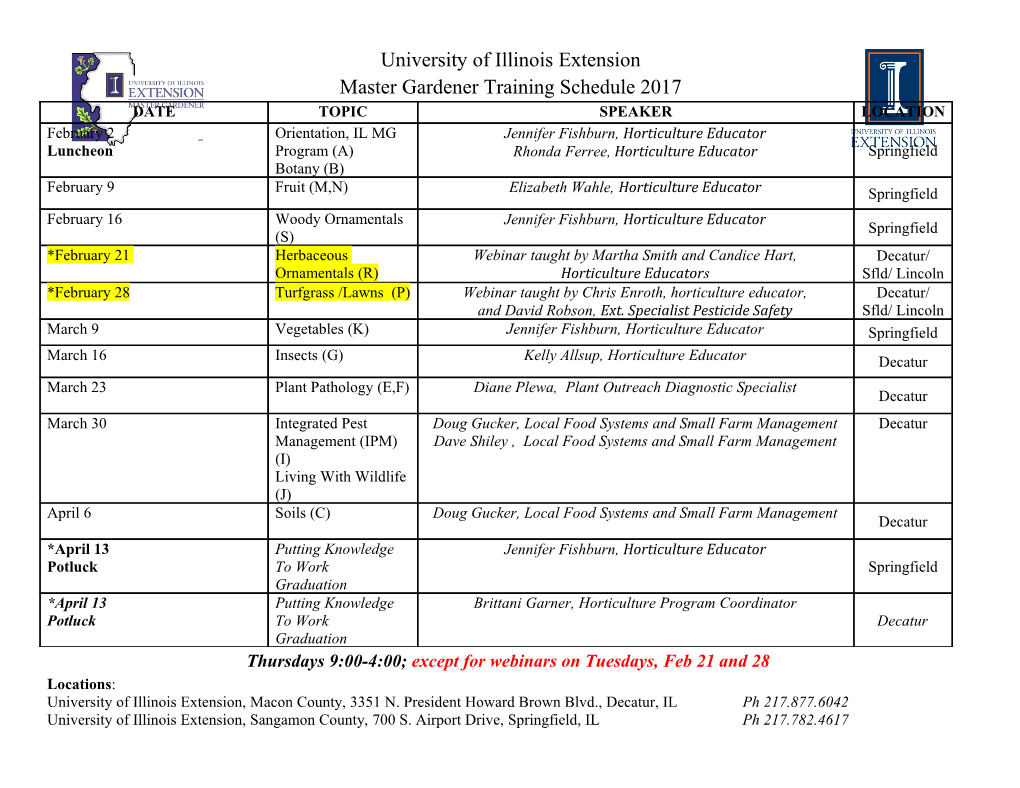
How a Scientific Discovery Is Made: A Case History The case of high-temperature superconductivity shows that discoveries have broad and deep root systems, hidden personal aspects, and lessons for science policy Gerald Holton, Hasok Chang and Edward Jurkowitz thoroughly investigate an informative A Brief History Even science in the has beenbest compared of times, to herd? managing case?the discovery of high-tempera? Superconductivity?the loss of electri? ing cats; it is not done well, but one is ture superconductivity?which, hav? cal resistance below a critical, or transi? surprised to find it done at all. In these ing occurred just 10 years ago, is recent tion, temperature (Tc) characteristic of days of diminishing resources, the enough to permit reconstructing its the material?was first discovered in analogy seems even more striking. Set? context. Our investigation, which in? mercury by the Dutch physicist Heike ting priorities for research, choosing cluded interviews with Karl Alex Kamerlingh Onnes in 1911. Mercury be? which projects are to be supported and M?ller and Johannes Georg Bednorz, comes superconducting at just 4.2 de? which abandoned, triggers epic battles the physicists who discovered the first grees above absolute zero (4.2 degrees at the highest levels. It requires pre? high-temperature superconductors, Kelvin). Teams large and small worked dicting which paths and which mix? throws light on a set of problems of in? for decades in the hope of finding elec? ture of policies might best advance sci? tense current interest: How does "cu? trical conductors with higher critical ence and lead to fruitful technologies. riosity-driven/' or basic, science interact temperatures, which would be easier Yet, in such debates, little attention is with "strategic" research and engineer? and cheaper to keep resistance-free. given to one of the most fundamental ing? How important are both planning There beckoned the rewards of both questions: What can historical cases and serendipity in discovery? What new theories to explain the phenome? teach us about what it takes to make a laboratory culture makes success more non and of practical applications to ex? scientific discovery? There are many likely? How deeply are the roots of ploit it; among the latter was the possi? popular ideas abroad, often based on crucial ideas and apparatus buried in bility of enormous new efficiencies in oversimplified textbook accounts of fa? the soil of history? How important is the transmission and use of electricity. mous discoveries and on charming the practice of borrowing across tradi? But nature, for a long time, yielded anecdotes. They have little to do with tions and disciplines? What role do the little hope for real progress. By 1973, the unruly complexity of the events private style and presuppositions of fully 62 years after the discovery of the themselves, and can only mislead sci? the individual play in research? phenomenon of superconductivity, all ence policymakers. Our study demonstrated that scien? efforts had stalled at a Tc of 23.3 Kelvin, For this reason, the authors of this tific innovation depends on a mixture the critical temperature of a niobium article welcomed an opportunity to of basic and applied research, on inter? germanium compound (Nb3Ge). After disciplinary borrowing, on an unforced years of frustrating failures to boost Tc pace of work and on personal motiva? into a region where there were realistic Gerald Holton is Mallinckrodt Professor of tions that lie beyond the reach of the prospects for commercial use, high Physics and professor of the history of science at Harvard University. His most recent book, administrator's rule book. While many temperature superconductivity was no Einstein, History and Other Passions, deals of these findings may be familiar to longer considered a promising area. with the perennial fight over the place given to sci? students of scientific creativity, our op? Some theories held that no higher Tc ence in our culture. Hasok Chang is a lecturer in erational mode of analysis, which is could be expected. Bernd Matthias, a the philosophy of science at University College, roughly comparable to the methods of highly respected Bell Laboratories University of London. His main research interests genealogical research, makes them physicist who, together with collabo? include the history and philosophy of modern more precise, testable and generally rators, had discovered hundreds of physics, and the philosophical analysis of measure? ment methods in various sciences. Edward applicable. As such they may serve as new superconductors, challenged his an empirical complement to some of peers to give up "theoretically motivat? Jurkowitz, a postdoctoral fellow, is currently at the the untested assumptions that inform ed" searches, or "all that is left in this Max Planck Institute f?r Wissenschaftgeschichte in Berlin, studying the history of quantum theory. policy discussions, especially the an? field will be these scientific opium ad? guished debates in Washington and in dicts, dreaming and reading one an? Address for Holton: Jefferson Physical Laboratory, other's absurdities in a blue haze" Harvard University, Cambridge, MA 02138. corporate boardrooms over the relative Internet: [email protected]. merits of applied and basic research. (quoted in Bromberg 1995). 364 American Scientist, Volume 84 This content downloaded from 140.247.137.37 on Mon, 29 Jul 2019 20:22:04 UTC All use subject to https://about.jstor.org/terms Figure 1. Many of the elements that underlie a scientific discovery can be identified by tracing citations in published articles, but ideas also come from outside the realm of science. In the case of Karl Alex M?ller's work, as the authors will explain later in this article, a major motiva? tion was the scientist's feeling that the highly symmetrical crystal structure that proved essential to the discovery of high-temperature supercon? ductivity had the affective power of a mandala, the highly symmetrical visual symbol of the universe used in Hinduism and Buddhism as a guide to meditation. M?ller later chose the Dharmaraja mandala, above, to illustrate an introspective essay on his sources of inspiration. All this changed practically overnight (La2Cu04 or lanthanum cuprate, measure the phenomenon had been in 1986, with the publication of a set of doped with a small amount of bari? available for decades. papers by Karl Alex M?ller and his for? um). Not only did it have a remark? The discovery became an academic mer student Johannes Georg Bednorz, ably high Tc?in the neighborhood of and popular sensation, especially after two investigators at the IBM Zurich Re? 30 Kelvin?it was relatively easy to Paul C. W. Chu's group at the Universi? search Laboratory in R?schlikon, Swit? prepare by ceramic techniques and to ty of Houston and Mao-Ken Wu's team zerland. Unlike most of the previously modify by chemical substitution. at the University of Alabama jointly an? discovered superconductors, the new Whereas ground-breaking discoveries nounced in February 1987 that they had compound was a ceramic, a mixed ox? often involve new technology, in this achieved superconductivity at about 90 ide of barium, lanthanum, and copper instance the means to create and to Kelvin with materials related to the 1996 July-August 365 This content downloaded from 140.247.137.37 on Mon, 29 Jul 2019 20:22:04 UTC All use subject to https://about.jstor.org/terms tures well above 200 Kelvin (the freez? ing point of water is 273.16 K). The opi? um addicts' blue haze has dissipated, and some physicists have even dared to hope again that room-temperature su? perconductors will eventually be found. A First-Level Analysis The main outlines of this discovery are well known, but our interviews and correspondence with M?ller and Bed norz turned up essential details miss? ing from other accounts. Here we set forth an account of the discovery of the first high-temperature superconduc? tors as M?ller and Bednorz experi? enced it, with particular attention to the resources, either intellectual or ma? terial, on which the discovery depend? ed. Then, based on this narrative, we put forward a systematic analysis, a schema designed to help answer the more general question of what it takes to make a scientific advance. M?ller, who was born in Basel, Switzerland, in 1927, graduated from the Swiss Federal Institute of Technolo? gy (ETH) in Zurich in 1958. ETH was the home base of the physicist Wolf? gang Pauli, who continued to teach Figure 2. Superconductivity, the loss of electrical resistance in a material, was discovered by the Dutch physicist Heike Kamerlingh Onnes (right, shown with colleague G. J. Flim and there after winning the Nobel prize in their helium-liquefying apparatus in 1922). Onnes found that mercury becomes supercon? 1945. M?ller said of Pauli, "he formed ducting at 4.2 degrees Kelvin (above absolute zero). Until Karl Alex M?ller and Georg and impressed me." As we were to dis? Bednorz published papers in 1986 describing a material that becomes superconducting at 30 cover, the student had learned more Kelvin, the discovery of superconductivity appeared to have little practical use. Onnes is than physics from his teacher. By 1963, only one of a large number of scientific "ancestors" to M?ller and Bednorz. (Photograph M?ller had joined the research staff of courtesy of the Deutsches Museum, Munich.) the IBM Zurich Research Laboratory, and in 1972 he was put in charge of the Bednorz-M?ller compound, a tempera? peak in Darien, the physicists at the physics group there. In 1982 he was ture well within the range of the inex? meeting "look'd at each other with a promoted to IBM Fellow, becoming pensive coolant liquid nitrogen.
Details
-
File Typepdf
-
Upload Time-
-
Content LanguagesEnglish
-
Upload UserAnonymous/Not logged-in
-
File Pages12 Page
-
File Size-