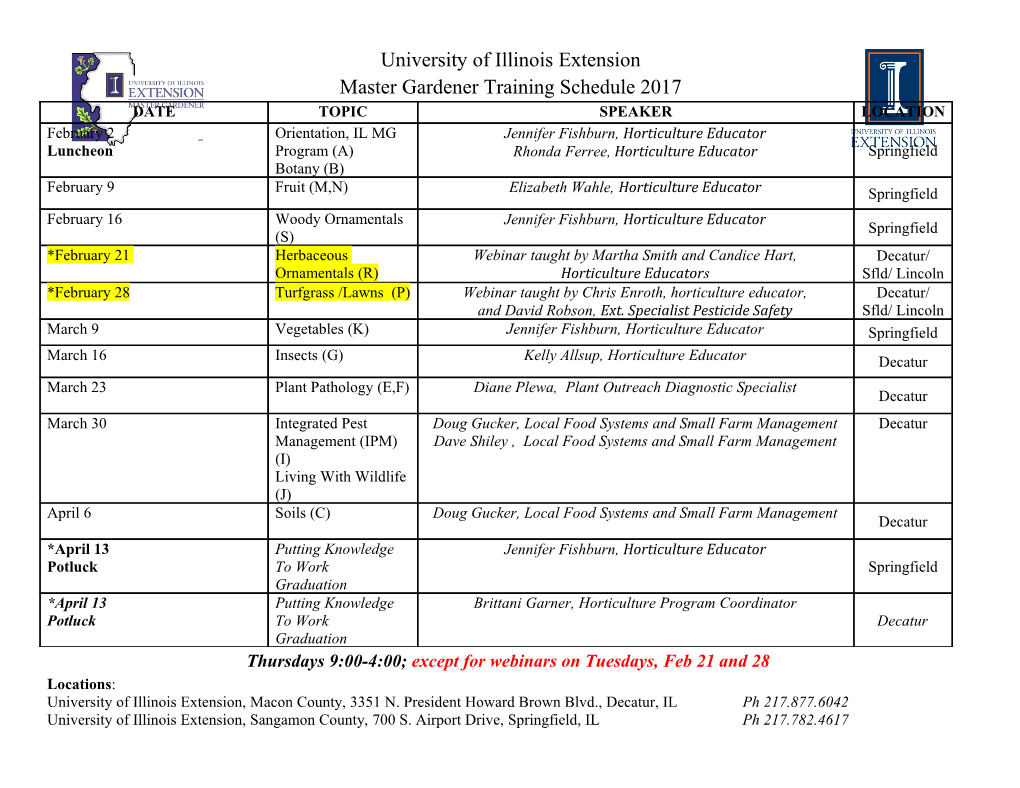
5 Habitable zones and Planetary atmospheres 5.1 Introduction Questions to answer: How does star affect the emergence and sustainability of life on a planet? What are the main properties of planetary atmospheres in the Solar system? How and what can we learn about atmospheres of extrasolar planets? 5.2 Habitable zones Unique Earth’s properties enabling the life support: liquid water planetary environment (atmosphere, etc.) A circumstellar habitable zone is defined as encompassing the range of distances from a star for which liquid water can exist on a planetary surface. Pure water exists as a liquid between 273 K and 373 K, unless the pressure is too low. Therefore, the primary factor in determining a planet’s habitability is temperature. The planet temperature is defined by the balance between absorption of stellar radiation (neglecting internal sources) re-radiation of it The stellar energy absorbed by the planet is L ER 2 * (1 a ) , ap4 d 2 where L* is stellar luminosity, Rp is radius of the planet, d is distance between the planet and the star, and a is albedo. The amount of energy to be radiated by the planet can be expressed as 24 Lp 4 RTpe , where Te is effective temperature of the planet. The balance between Lp and Ea defines the effective temperature of the planet. The effective temperature of the Earth is 255 K. It is lower than 273 K because the Earth is not a perfect black body and it traps some energy because of the greenhouse effect . If the Sun would be more luminous, to maintain the same effective temperature the Earth should be further away, and vice versa: Astrobiology: 5. Habitable zones and Planetary Atmospheres 5-1 S.V. Berdyugina, University of Freiburg Figure 1: Left: the distance to the habitable zone versus stellar luminosity calculated from the balance between Lp and Ea. Right: the evolutionary track of a solar-type star starting on the Main Sequence and continued through the Red Giant and Horizontal Branch phases to the Asymptotic Giant Branch. The luminosity of the star increases by several orders of magnitude. 4 Gyr ago the solar luminosity was lower by 30%, as main-sequence stars move up along the sequence and further to the red giant phases habitable zone was closer to the Sun habitable zone is moving away from the Sun The continuous habitable zone (CHZ) is the region, in which a planet may reside and maintain liquid water throughout most of a star’s life. Figure 2: Continuous habitable zone: yellow region is the habitable zone in the beginning of the main sequence, blue region is the habitable zone at late stages of the stellar evolution, and the green region is an intersection of the other two. Astrobiology: 5. Habitable zones and Planetary Atmospheres 5-2 S.V. Berdyugina, University of Freiburg Figure 3: Evolution of the solar luminosity (left) and the HZ (right). The most realistic model of the HZ is depicted by long dashes (Kasting et al. 1993). To estimate realistically the CHZ for the Sun, one needs to take into account albedo greenhouse effect The inner edge of the CHZ is determined by photodissociation of water and loss of hydrogen. 0.95 AU The outer edge of the CHZ is mainly defined by formation of CO2 clouds. 1.15 AU This contradicts however to the fact that surface of Mars was once carved by streams of some flowing liquid. The used climate model should be modified by adding other greenhouse gases (e.g. CH4) and perhaps by including a more dense cloud cover on Mars. Tidal heating of satellites around giant planets, such as Jupiter’s satellite Europa, raises the possibility of liquid water existing below the surface of ice-covered satellites. Sun in time To understand the early phase of the planet formation and the evolution of the habitable zone in the Solar system, we need to study the effects of the young Sun on planets. This is possible with a sample of stars of about the same mass as the Sun but at different evolutionary stages. A study of such stars shows that the solar radiation has undergone dramatic variations since it arrived to the Main Sequence. For instance, the X-ray luminosity of the young Sun (~108 years) was 1000 times of the present level. Fig. 4 shows the X-ray luminosity of solar-type stars versus the age. In Fig. 5 this is compared with the radiation in other wavelengths. Astrobiology: 5. Habitable zones and Planetary Atmospheres 5-3 S.V. Berdyugina, University of Freiburg Figure 4: The X-ray luminosity of solar-type stars versus the age. Note the decrease by three orders of magnitude over 10 Gyr. Figure 5: The X-ray, UV and total luminosity of the Sun versus age as deduced from solar analogues. The young Sun had UV radiation factor of 10 to 100 higher than the present level, while its total luminosity was in fact only 70% of the present. Magnetic activity of the Sun (flares, coronal mass ejections, solar wind, etc.) was also much more prominent as compared to the Sun today. These factors have significantly influenced the planetary atmospheres, their composition and density. Astrobiology: 5. Habitable zones and Planetary Atmospheres 5-4 S.V. Berdyugina, University of Freiburg Effects of Solar magnetic activity on terrestrial planets Mercury 0.39 AU from the Sun Variation of the mean density with diameter of the terrestrial planets as well as the Moon (shown on the right) indicates that Mercury has a much higher mean density than expected given its size. This is due to the relative size of its iron core which is significantly larger than for any other terrestrial planet. One possible explanation is that Mercury’s lighter mantle/crust was eroded away by the strong (~1,000 times present values) winds and the early Sun’s higher extreme ultraviolet fluxes. The eroded and ionized material has been carried away with the solar wind. Figure 6: The mean density of terrestrial planets versus the diameter. Mercury significantly deviates from the common tendency. Venus 0.71 AU from the Sun No water or oxygen. The explanation is in photochemistry/photoionization effects: Venus has a slow rotation period (P= 243 days) and a very weak magnetic dynamo Venus is thus not protected from the Sun’s plasma by planetary magnetic field Perhaps the young Sun’s enhanced activity and UV flux played a major role: e.g. Photodissociation of water H2O H + OH, and subsequent loss of H. Earth 1 AU from the Sun A Young active Sun may have played a major role in the evolution of the Earth’s atmosphere and possibly the origin and evolution of life. Destruction of methane (CH4) by the early Sun’s strong UV radiation Formation of ozone (O3) via photoionization of O2 Photochemical reactions leading to the formation of organic molecules Erosion of the atmosphere due to enhanced solar wind: loss of H+, O++, N+ Astrobiology: 5. Habitable zones and Planetary Atmospheres 5-5 S.V. Berdyugina, University of Freiburg Mars 1.52 AU from the Sun Today, Mars is a cold dry planet with a thin atmosphere rich in CO2. Mars also possesses a very weak magnetic field. There is also geological evidence of running water and possibly a permanent layer of permafrost. It is important to study the effects of the active young Sun on Mars: Loss of water and atmosphere Soil oxidation Possible early life Early Mars: liquid iron core produced a magnetic field strong enough to protect the young Martian atmosphere and surface water from the punishing effects of the young Sun’s intense solar wind. (Lammer et al. 2003). Roughly 3.5 Billion years ago, Mars’ core solidified, shutting down the Martian magnetic dynamo. Consequences: Without a magnetic field, the outer Martian atmosphere was subjected to the ionizing effects and strong winds of the sun, and began to erode. At this time, water disassociates into 2H+O, where the lighter hydrogen is lost to the space while the heavier oxygen combines with iron on its surface Habitable zones around other stars In principle low-mass stars (dwarfs) are prime candidates for searches of planets in habitable zones: Long life times on the Main Sequence (>10Gyr, see figure below) Very abundant in the solar neighborhood (>70%) Better contrast star/planet for detection 4 1.4 M.; F4-51.4 M 1.0 M.;1.0 G2 M 3 F 4-5 G2 2 “stable” lifetime ~2.5 Gyr “stable” lifetime 1 L/L . Figure 7: Variations of ~8 Gyr the total luminocity for log stars of different masses 0 and spectral classes. “stable” lifetime >20 Gyr 0.70.7 M M.;, K K2-3 2-3 Low-mass K-M dwarfs have long periods of -1 “stable” lifetime >40 Gyr 0.4 M, M 1-2 constant luminosity and 0.4 M.; M1-2 are therefore prime -2 candidates for searches 0.0 5.0e+9 1.0e+10 1.5e+10 2.0e+10 of planets in habitable Age (years) zones. Astrobiology: 5. Habitable zones and Planetary Atmospheres 5-6 S.V. Berdyugina, University of Freiburg Stars later than F0 have main sequence lifetimes exceeding 2 Gyr. They are therefore potential candidates for harboring habitable planets. The HZ around an F star is larger and occurs farther out than for our Sun. The CHZ of F stars are narrower (log distance) than for the Sun because they evolve more rapidly. the HZ around K and M stars is smaller and occurs closer to the star.
Details
-
File Typepdf
-
Upload Time-
-
Content LanguagesEnglish
-
Upload UserAnonymous/Not logged-in
-
File Pages18 Page
-
File Size-