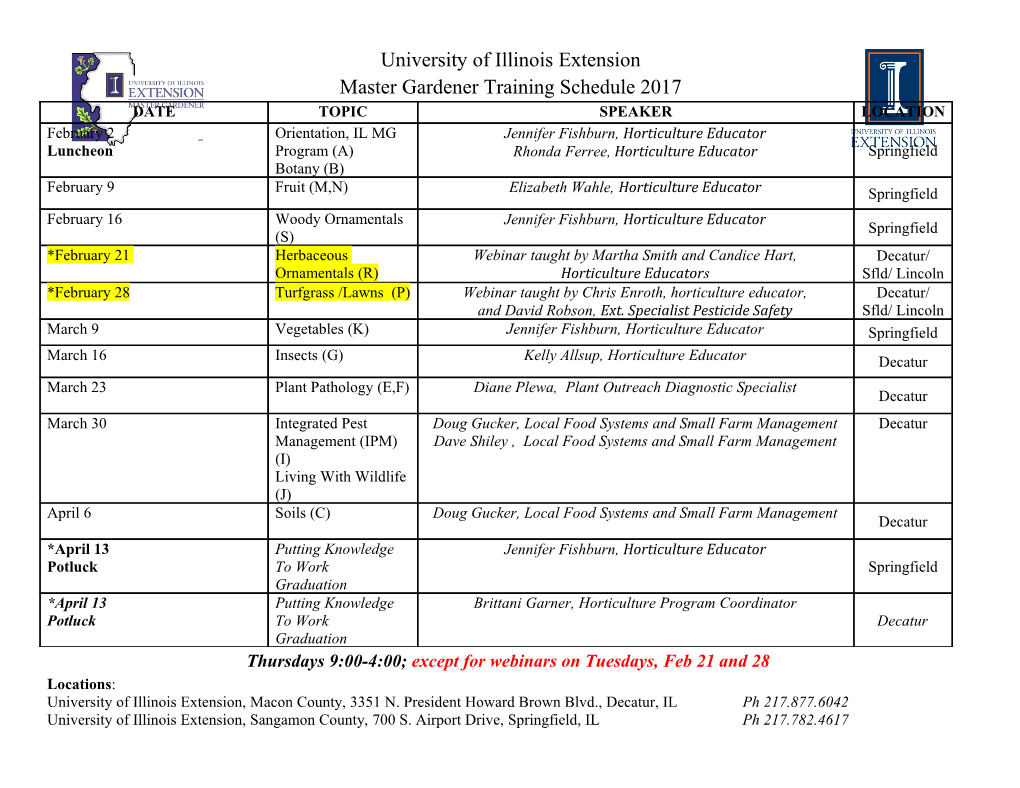
Quantum metamaterial for nondestructive microwave photon counting 1;2 1;3 4;5 6;7 6;7 4;5 Arne L. Grimsmo∗ , Baptiste Royer , John Mark Kreikebaum , Yufeng Ye , Kevin O'Brien , Irfan Siddiqi , Alexandre Blais1;8 1 Institut quantique and D´epartment de Physique, Universit´ede Sherbrooke, Sherbrooke, Qu´ebec, Canada J1K 2R1 2 Centre for Engineered Quantum Systems, School of Physics, The University of Sydney, Sydney, NSW 2006, Australia 3 Department of Physics, Yale University, New Haven, CT 06520, USA 4 Materials Science Division, Lawrence Berkeley National Laboratory, Berkeley, California 94720, USA 5 Quantum Nanoelectronics Laboratory, Department of Physics, University of California, Berkeley, California 94720, USA 6 Research Laboratory of Electronics, Massachusetts Institute of Technology, Cambridge, MA 02139, USA 7 Department of Electrical Engineering and Computer Science,Massachusetts Institute of Technology, Cambridge, MA 02139, USA 9 Computational Research Division, Lawrence Berkeley National Laboratory, Berkeley, California 94720, USA 8 Canadian Institute for Advanced Research, Toronto, Canada Detecting traveling photons is an essential prim- the detection of dark matter axions [20]. itive for many quantum information processing A number of theoretical proposals and experimental tasks. We introduce a single-photon detector de- demonstrations of microwave single-photon detectors have sign operating in the microwave domain, based on emerged recently. These schemes can broadly be divided a weakly nonlinear metamaterial where the nonlin- into two categories: Time-gated schemes where accurate earity is provided by a large number of Josephson information about the photon's arrival time is needed a junctions. The combination of weak nonlinearity priori [2, 6, 7, 10, 11, 13], and detectors that operate con- and large spatial extent circumvents well-known tinuously in time and attempt to accurately record the obstacles limiting approaches based on a localized photon arrival time [1, 3, 4, 5, 8, 9, 12, 13]. In this work, Kerr medium. Using numerical many-body simu- we are concerned with the last category, which is simul- lations we show that the single-photon detection taneously the most challenging to realize and finds the fidelity increases with the length of the metama- widest range of applications. terial to approach one at experimentally realistic Depending on the intended application, there are sev- lengths. A remarkable feature of the detector is eral metrics characterizing the usefulness of single-photon that the metamaterial approach allows for a large detectors. Not only is high single-photon detection fi- detection bandwidth. In stark contrast to conven- delity required for many quantum information applica- tional photon detectors operating in the optical tions, but large bandwidth, fast detection and short dead domain, the photon is not destroyed by the de- times are also desirable [21]. Moreover, nondestructive tection and the photon wavepacket is minimally photon counting is of fundamental interest and offers new disturbed. The detector design we introduce of- possibilities for quantum measurement and control. In fers new possibilities for quantum information pro- this article, we introduce the Josephson Traveling-Wave cessing, quantum optics and metrology in the mi- Photodetector (JTWPD), a non-destructive single-photon crowave frequency domain. detector which we predict to have remarkably high perfor- mance across the mentioned metrics. In particular, this detector can have detection fidelities approaching unity Introduction without sacrificing detector bandwidth. In contrast to infrared, optical and ultraviolet frequencies The JTWPD exploits a weakly nonlinear, one- dimensional metamaterial, designed to respond to the arXiv:2005.06483v1 [quant-ph] 13 May 2020 where single-photon detectors are a cornerstone of experi- mental quantum optics, the realization of a detector with presence of a single photon. The nonlinearity is provided similar performance at microwave frequencies is far more by a large number of Josephson junctions, inspired by the challenging [1, 2, 3, 4, 5, 6, 7, 8, 9, 10, 11, 12, 13]. The Josephson traveling wave parametric amplifier [22]. Be- interest in realizing such a detector is intimately linked cause the detector response does not rely on any resonant to the emergence of engineered quantum systems whose interaction, the detector bandwidth can be designed to natural domain of operations is in the microwaves, includ- range from tens of MHz to the GHz range. The detec- ing superconducting quantum circuits [14], spin ensem- tion and reset times are predicted to be in the range of bles [15], and semiconductor quantum dots [16]. The con- tens of µs for typical parameters. Moreover, the signal- tinuing improvement in coherence and control over these to-noise ratio (SNR) grows linearly with the length of the quantum systems offers a wide range of new applications metamaterial which can be made large, leading to single- for microwave single-photon detection, such as photon- photon detection fidelities approaching unity. By interro- based quantum computing [17], modular quantum com- gating the nonlinear medium with a \giant probe" [23]|a puting architectures [18], high-precision sensing [19], and probe system that couples to the medium over a spatial extent that is large compared to the length of the signal ∗Corresponding author. Email: [email protected] photons|this approach bypasses previous no-go results 1 of coupled LC oscillators, in a configuration known as composite right/left handed (CRLH) metamaterial [28]. The LC oscillators are coupled via an array of nonlinear couplers (inset) to a readout resonator acting as a giant probe (blue). With the metamaterial coupled at x = z=2 to impedance matched linear transmission lines, the inter-± action time between the photon and the giant probe is τ = z=v where v is the speed of light in the metamaterial. Figure 1: a) Sketch of the JTWPD. Standard trans- As an alternative to this transmission mode, the interac- mission lines (black) are coupled to both ends of a one- tion time can be doubled by terminating the metamaterial dimensional metamaterial (orange) of length z and linear at x = +z=2 with an open where the photon wavepacket is dispersion relation, ! = vk. A cross-Kerr interaction χ be- reflected. To simplify the analysis, we consider the trans- tween the metamaterial and the giant probe mode (blue) mission mode in most of the treatment below, but return leads to a phase shift in the strong measurement tone to a discussion of reflection mode when discussing poten- (yellow) while the signal photon (red) travels through the tial experimental implementation and parameters. ^ metamaterial. b) Phase space picture of the probe mode. The full detector Hamiltonian can be expressed as H = ^ ^ ^ ^ With respect to the idle coherent state α , the presence of H0 + Hr + Hint, where H0 contains the linear part of the a signal photon displaces the states by jgz=vi , with g = χα. waveguide including the metamaterial as well as the input and output linear waveguides, H^r is the probe resonator Hamiltonian and H^int describes the nonlinear coupling be- for photon counting based on localized cross-Kerr interac- tween the probe and the metamaterial. As shown in the tions [24, 25, 26, 27]. Supplementary Materials, in the continuum limit where the size a of a unit cell of the metamaterial is small with respect to the extent of the photon wavepacket, H^0 takes Results the form ^ ^ ^ H0 = d!~!bν!y bν!: (1) Many proposals for itinerant microwave photon detection ν= ZΩ rely on capturing the incoming photon in a localized ab- X± ^ In this expression, by ! creates a delocalized right/left- sorber mode that is interrogated using heterodyne de- ± tection [1, 3, 4, 5, 12, 13]. A first challenge associated moving photon with energy ~! and satisfies the canoni- cal commutation relation [^bν!; ^by ] = δνµδ(! !0). The with this approach is linked to a version of the quantum µω0 − Zeno-effect: continuously and strongly monitoring the ab- subscript Ω in Eq. (1) is used to indicate that we only sorber will prevent the incoming photon from being ab- consider a band of frequencies around which the metama- sorbed [1, 12], limiting the detector's quantum efficiency. terial's dispersion relation is approximately linear. The ^ Another difficulty concerns the tradeoff between efficiency probe resonator Hamiltonian Hr can be written in a dis- and bandwidth. A large detector response to a single pho- placed and rotating frame with respect to the coherent ton requires a sufficiently long interaction time with the drive field as ~K 2 2 photon. In principle, this can be achieved by making the H^ 0 = a^y a^ ; (2) r 2 absorber mode long-lived. However, as the mode linewidth is inversely proportional to the photon lifetime, this im- where K is a self-Kerr nonlinearity induced by the nonlin- poses a serious constraint on the detector bandwidth. ear couplers (see Methods). Our solution to overcome these obstacles is illustrated The coupling elements also lead to cross-Kerr interac- schematically in Fig. 1: In place of a localized absorber, we tion between the array of oscillators and the probe mode. use a long and weakly nonlinear metamaterial. Backscat- As mentioned above, this coupling is chosen to be locally tering is avoided by using a nonlinearity that is locally weak such that the nonlinearity is only activated by the weak, yet a large response is made possible by having a presence of a strong coherent drivea ^in(t) on the probe. In ^ long photon time-of-flight through the metamaterial. The this limit, the nonlinear interaction Hamiltonian Hint is in presence of a photon is recorded using a continuously mon- the same rotating and displaced frame given by itored probe mode that is coupled to the metamaterial z=2 ^ ^ ^ 2 along the full extent of its length. Thanks to a nonlinear Hint0 = ~ dxχ(x)bνy (x)bµ(x) a^ya^ + α z=2 cross-Kerr coupling, in the presence of the measurement νµ Z− X (3) tonea ^in(t), a single photon in the metamaterial induces a z=2 ^ ^ displacement of the output fielda ^out(t) relative to its idle + ~ dxg(x)bνy (x)bµ(x) a^y +a ^ ; z=2 state.
Details
-
File Typepdf
-
Upload Time-
-
Content LanguagesEnglish
-
Upload UserAnonymous/Not logged-in
-
File Pages45 Page
-
File Size-