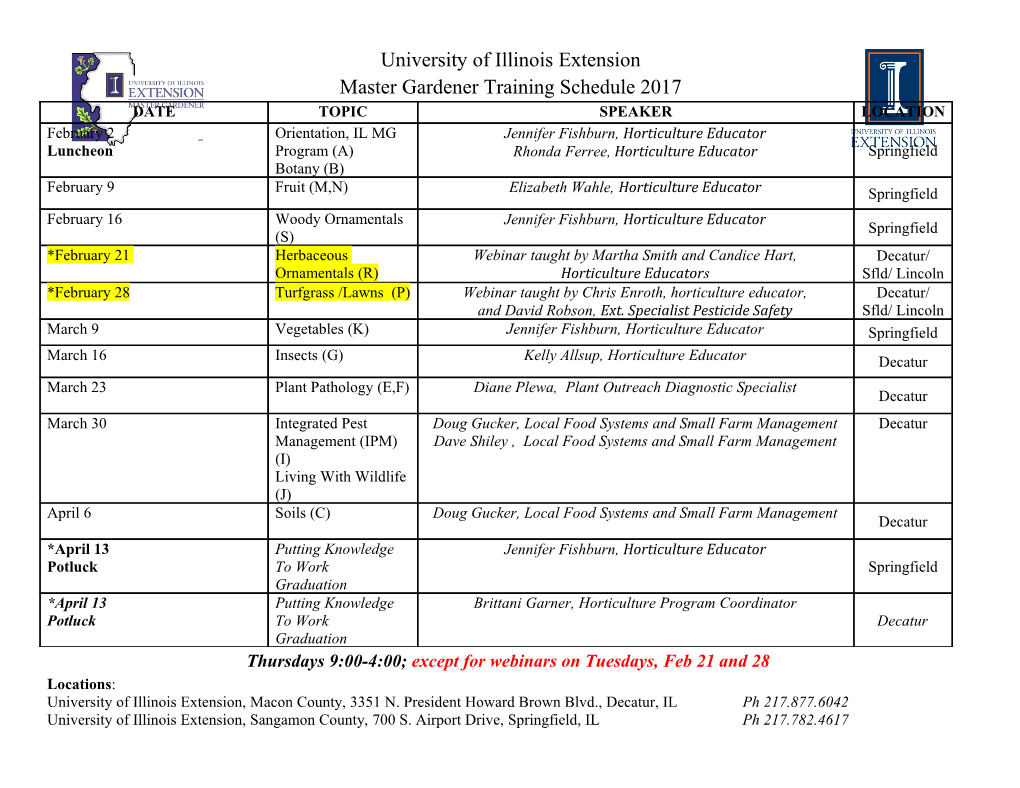
Lecture Notes 1 Silicon Photodetectors • Light Intensity and Photon Flux • Photogeneration in Silicon • Photodiode ◦ Basic operation ◦ Photocurrent derivation ◦ Quantum efficiency ◦ Dark current • Direct Integration • Photogate • Appendices ◦ Appendix I: Derivation of Continuity Equation ◦ Appendix II: Depletion Width for PN Junction ◦ Appendix III: MOS Capacitor ◦ Appendix IV: Useful Data EE 392B: Silicon Photodetectors 1-1 PSfrag replacements Preliminaries • Photodetector is the front end of the image sensor. It converts light incident on it into photocurrent that is (hopefully) proportional to its intensity • Conversion is done in two steps: ◦ Incident photons generate e-h pairs in the detector (e.g., silicon) ◦ Some of the generated carriers are converted into photocurrent • Photocurrents are typically very small (10s to 100s of fA) ◦ Direct measurement is difficult ADC ◦ Usually integrated into charge on a capacitor and then converted to Gain voltage before readout DN Photonflux Current density Charge Voltage Quantum Efficiency Integration Conversion space/time Gain ph/cm2·sec A/cm2 Col V EE 392B: Silicon Photodetectors 1-2 Visible Light • We are mainly concerned with visible light image sensors • Recall that the energy of a photon is given by Eph = hc/λ, where h = 4:135 × 10−15eV.sec is Planck's constant, c = 3 × 108m/s is the speed of light, and λ is the wavelength • Visible light wavelengths (λ) range from 400 nm to 700 nm Violet: 400 nm (Eph = 3:1 eV) Blue: 450 nm (Eph = 2:76 eV) Cyan: 500 nm (Eph = 2:48 eV) Green: 550 nm (Eph = 2:27 eV) Yellow: 600 nm (Eph = 2:08 eV) Red: 700 nm (Eph = 1:77 eV) Infrared: > 800 nm (Eph < 1:55 eV) EE 392B: Silicon Photodetectors 1-3 • The amount of light incident on an image sensor surface depends on ◦ The light source ◦ The surface reflectance of the object being imaged ◦ The imaging optics used • Different visible light sources, e.g., daylight (D65), incandescent, halogen, fluorescent have different power spectra EE 392B: Silicon Photodetectors 1-4 Radiometry and Photometry • Two ways to measure the intensity of light incident on a surface: ◦ Radiometry measures it as irradiance E W/m2 2 ◦ Photometry measures it as illuminance Eν in lux or lumens/m , which 1 2 is defined as 683W/m at λ = 555nm • Illuminance takes into account the sensitivity of the human eye to different wavelengths; λ = 555nm is the wavelength for which the human eye is most sensitive and the value for which the photopic vision curve is normalized 1 0.9 0.8 0.7 0.6 0.5 0.4 0.3 relative sensitivity 0.2 0.1 0 350 400 450 500 550 600 650 700 750 wavelength [nm] EE 392B: Silicon Photodetectors 1-5 • Translating from irradiance to illuminance: Denote the vision photopic curve as Y (λ) and the irradinace density E(λ) W/m2.nm, then the illuminance is given by 700 Eν = 683 Y (λ)E(λ) dλ lux Z400 EE 392B: Silicon Photodetectors 1-6 Photon Flux 2 • Photon flux F0 is the number of photons per cm .sec incident on a surface • Using the photon energy Eph(λ), we can readily translate irradiance density E(λ) into photon flux 700 10−4E(λ) F = dλ photons/cm2.sec 0 E (λ) Z400 ph • Translating from illuminance to photon flux: −20 ◦ At λ = 555nm, Eph = 35:8 × 10 Joule; thus 1 lux corresponds to 16 11 2 F0 = 10 =683 × 35:8 = 4:09 × 10 photons/cm ·sec, or, 133 photons strike a 1µm × 1µm surface per 1/30 sec ◦ A typical light source (e.g., D65) has a wide range of wavelengths 12 2 and 1 lux roughly corresponds to F0 ≈ 10 photons/cm .sec, or, 333 photons strike a 1µm× 1µm surface per 1/30 sec EE 392B: Silicon Photodetectors 1-7 • Photon flux values encountered vary over a very wide range: 4 17 clear sky ≈ 10 Lux, or F0 = 10 13 room light ≈ 10 Lux, or F0 = 10 11 full moon ≈ 0:1 Lux, or F0 = 10 −4 8 moonless night ≈ 10 Lux, or F0 = 10 EE 392B: Silicon Photodetectors 1-8 Photocharge Generation in Semiconductors • Incident photon energy must be > band gap energy (Eg) to generate an electron-hole pair ◦ Electrons go to the conduction band (EC) ◦ Holes go to the valence band (EV ) • Energy band diagram of silicon: Ec PSfrag replacements Eg = 1:124eV Ev • Coincidentally (and luckily) photons in the visible range have enough energy to generate e-h pairs ◦ No photon can generate more than one e-h pair • Energy gap of other semiconductors: Ge (0.66 eV), GaAs (1.42 eV) EE 392B: Silicon Photodetectors 1-9 Photocharge Generation Rate in Silicon 2 • Assume a monochromatic photon flux F0 photons/cm .sec at wavelength λ incident at the surface (i.e., x = 0) of silicon photon flux 0 x PSfrag replacements silicon e-h pair • The photon absorption in a material is governed by its absorption coefficient α(λ) cm−1 • Let F (x) be the photon flux at depth x, then the number of photons absorbed per second between x and x + ∆x is given by F (x) − F (x + ∆x) ≈ αF (x)∆x; EE 392B: Silicon Photodetectors 1-10 We can write this equation in the limit as dF (x) = −αF (x) dx Solving we obtain −αx 2 F (x) = F0e photons/cm .sec Thus the rate of e-h pairs generated at x is d G(x) = (F − F (x)) = αF e−αx e-h pair/cm3.sec dx 0 0 EE 392B: Silicon Photodetectors 1-11 Absorption Coefficient of Silicon 7 10 6 10 ] 1 − 5 10 [cm 4 10 Cofficient 3 10 rption Abso 2 10 PSfrag replacements 1 10 200 300 400 500 600 700 800 900 1000 Wavelength [nm] E. Palik, "Handbook of Optical Constant of Solids," Academic, New York, 1985 EE 392B: Silicon Photodetectors 1-12 Absorption Length of Visible Light in Silicon EE 392B: Silicon Photodetectors 1-13 Light Absorption in a Silicon Slab EE 392B: Silicon Photodetectors 1-14 Comments • F (x) and G(x) are average values assuming a large ensemble of photons (approaching continuum values) ◦ The photon absorption process is actually discrete and random • Note that: ◦ 99% of blue light is absorbed within 0.6 µm ◦ 99% of red light is absorbed within 16.6 µm • These depths (surprisingly) are quite consistent with the junction and well depths of a CMOS process • But, this is not the whole story . ◦ Photocharge needs to be collected and converted into electrical signal EE 392B: Silicon Photodetectors 1-15 Photodetectors in Silicon • A photodetector is used to convert the absorbed photon flux into photocurrent • There are three types of photodetectors used, photodiode, which is a reverse biased pn junction, photogate, and pinned diode • In a standard CMOS process there are three types of photodiodes available ◦ nwell/psub ◦ n+/psub ◦ p+/nwell and two types of photogates ◦ nMOS transistor gate to drain ◦ pMOS transistor gate to drain EE 392B: Silicon Photodetectors 1-16 • In this lecture notes we discuss the photodiode and photogate operation. The pinned diode will be discussed in the following lecture notes EE 392B: Silicon Photodetectors 1-17 Photodiode Operation • Assume the depletion approximation of a reverse biased pn junction PSfrag replacements photon flux quasi-neutral n-type n-region vD > 0 depletion region iph quasi-neutral p-region p-type • The photocurrent, iph, is the sum of three components: ◦ Current due to electrons generated in the depletion (space charge) sc region, iph p ◦ Current due to holes generated in the quasi-neutral n-region, iph n ◦ Current due to electrons generated in the quasi-neutral p-region, iph EE 392B: Silicon Photodetectors 1-18 • Most electrons generated in the depletion region are converted into current by strong electric field • Carriers generated in the quasi-neutral regions need to diffuse to the depletion region to be collected ◦ Some charge is lost through recombination ◦ The diffusion length determines the fraction of charge that is not recombined EE 392B: Silicon Photodetectors 1-19 Photocurrent Derivation • Assumptions ◦ Abrupt pn junction ◦ Depletion approximation ◦ Low level injection, i.e., flux induced carrier densities << majority carrier densities ◦ Short base region approximation, i.e., junction depths << diffusion lengths. This is is quite reasonable for advanced CMOS processes • Our results are inaccurate but will help us understand the dependence of iph on various device parameters References: • F. Van de Wiele, \Photodiode Quantum Efficiency," in P. G. Jespers, F. van de Wiele, M. H. White eds. \Solid State Imaging," p. 47, Noordhoff (1976). • J.C. Tandon, D.J. Roulston, S.G. Chamberlain, Solid State Electronics, vol. 15, pp. 669 { 685, (1972). • R.W. Brown, S.G. Chamberlain, Physica Status Solidi (a), vol. 20, pp. 675 { 685 (1973) EE 392B: Silicon Photodetectors 1-20 PSfrag replacements • Consider the depletion approximation for a reverse biased pn junction photon flux v 0 quasi-neutrali n-type n-region x1 depletion region x2 quasi-neutral p-region p-type x3 x 2 • Assume a monochromatic photon flux F0 photon/cm ·sec incident at the surface (x = 0), the e-h generation rate at depth x is given by −αx 3 G(x) = αF0e ph/cm .sec • Assuming all generated electrons in the space charge region are collected, the current density due to generation in the space charge region is sc −αx1 −αx2 2 jph = qF0(e − e ) A/cm ; where q = 1:6 × 10−19Col is the electron charge EE 392B: Silicon Photodetectors 1-21 • The current density due to generation in n-type quasi-neutral region, which is diffusion current (since there is no field in this region), is given by 0 p @pn(x) jph = −qDp @x x=x1 0 where pn is the photogenerated minority carrier (hole) density, and Dp is the diffusion constant of holes (in cm2/sec) • To find the current density due to generation in the n-type quasi-neutral 0 region, we first need to find pn(x).
Details
-
File Typepdf
-
Upload Time-
-
Content LanguagesEnglish
-
Upload UserAnonymous/Not logged-in
-
File Pages75 Page
-
File Size-