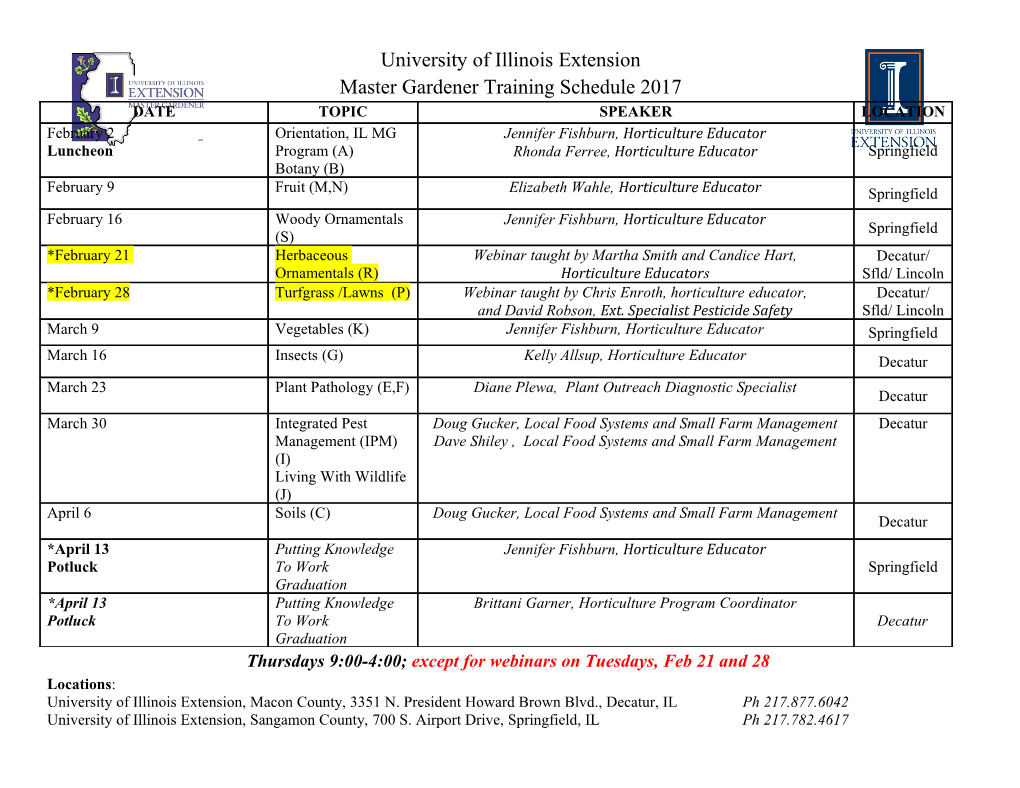
Alma Mater Studiorum – Università di Bologna DOTTORATO DI RICERCA IN CHIMICA Ciclo XXVIII Settore Concorsuale di afferenza: 03/C1 Settore Scientifico disciplinare: CHIM/06 Coupling reactions between aromatic carbon- and nitrogen- nucleophiles and electrophiles: reaction intermediates, products and their properties Presentata da: Silvia Cino Coordinatore Dottorato Relatore Prof. Aldo Roda Prof.ssa Carla Boga Co-relatore Dott. Gabriele Micheletti Esame finale anno 2016 TABLE OF CONTENTS INTRODUCTION : The aromatic substitution reactions 1 I. ELECTROPHILIC AROMATIC SUBSTITUTION REACTION (SEAR) 1 II. NUCLEOPHILIC AROMATIC SUBSTITUTION REACTION (S NAR) 5 6 III. SEAR/S NAR REACTIONS BETWEEN STRONGLY ACTIVATED NEUTRAL CARBON ELECTROPHILES AND NUCLEOPHILES IV. MAYR ’S ELECTROPHILICITY SCALE 11 REFERENCES 15 CHAPTER 1: Reaction between arenediazonium salts and neutral aromatic 17 carbon nucleophiles 1.1 AZO COUPLING BETWEEN AMINOTHIAZOLE DERIVATIVES AND ARENEDIAZONIUM 17 SALTS : NEW PRODUCTS AND UNEXPECTED LONG RANGE SUBSTITUTENTS TRANSMISSION EFFECT 1.1.1 Introduction 17 1.1.2 Results and Discussion 19 1.1.3 Conclusions 28 1.1.4 Experimental Section 28 1.2 REACTIONS BETWEEN ARENEDIAZONIUM SALTS AND SUBSTITUTED ANISOLE 33 DERIVATIVES : REACTIVITY , REGIOSELECTIVITY AND FORMATION OF SOLID STATE FLUORESCENT COMPOUNDS 1.2.1 Introduction 33 1.2.2 Results and Discussion 34 1.2.3 Conclusions 39 1.2.4 Experimental section 39 1.3 REACTIONS BETWEEN ARENEDIAZONIUM SALTS AND 1,3-DISUBSTITUTED ARENES 43 1.3.1 Introduction 43 1.3.2 Results and Discussion 43 1.3.3 Conclusions 48 1.3.4 Experimental section 48 1.4 NEW BENZIMIDAZOLE DERIVATIVES BY RING CLOSURE OF AZOCOMPOUNDS DERIVED 53 FROM 1,3,5-TRIS (DIALKYLAMINO )BENZENES AND ARYLDIAZONIUM SALTS 1.4.1 Introduction 53 1.4.2 Results and Discussion 55 1.4.3 Conclusions 59 1.4.4 Experimental section 60 REFERENCES 64 CHAPTER 2: SEAr/S NAr reactions between aromatic and heteroaromatic 67 neutral substrates 2.1 REACTIONS BETWEEN BENZOFUROXAN DERIVATIVES AND TRI -SUBSTITUTED ARENES 67 2.1.1 Introduction 67 2.1.2 Results and Discussion 68 2.1.3 Conclusions 72 2.1.4 Experimental section 73 2.2 NOVEL STRUCTURAL HYBRIDS FROM THE REACTION BETWEEN BENZOFUROXAN AND 77 BENZOTHIAZOLE DERIVATIVES 2.2.1 Introduction 77 2.2.2 Results and Discussion 78 2.2.3 Conclusions 85 2.2.4 Experimental section 86 2.3 REACTIONS OF CHLORO -NITRO -BENZOFURAZAN - AND BENZOFUROXAN - DERIVATIVES 93 WITH 1,3-BIS (N,N-DIALKYLAMINO )BENZENE DERIVATIVES 2.3.1 Introduction 93 2.3.2 Results and Discussion 93 2.3.3 Conclusions 98 2.3.4 Experimental section 99 REFERENCES 103 CHAPTER 3: New electron-withdrawing/donor architectures from 105 nitrothiophenes and 1,3,5-tris(dialkylamino)benzene derivatives 3.1 INTRODUCTION 105 3.2 RESULTS AND DISCUSSION 106 3.3 CONCLUSIONS 112 3.4 EXPERIMENTAL SECTION 113 REFERENCES 120 CHAPTER 4: Triaminobenzene derivatives versus benzhydrylium ions: 123 further evidence of the reversibility of the σσσ intermediates formation step in S EAr/S NAr reactions 4.1 INTRODUCTION 123 4.2 RESULTS AND DISCUSSION 124 4.3 CONCLUSIONS 135 4.4 EXPERIMENTAL SECTION 136 REFERENCES 144 INTRODUCTION The aromatic substitution reactions The Electrophilic and Nucleophilic aromatic substitution reactions have been extensively studied over the years [1,2] and their mechanism is well known and widely reported in the literature. I. ELECTROPHILIC AROMATIC SUBSTITUTION REACTION (SEAR) The first example of the S EAr concerns the substitution of a hydrogen atom on the benzene ring with an atom or group (indicated as E in Scheme1). Benzene is, in fact, the parent of the aromatic compounds; it has a very high thermodynamic stability due to the delocalization of its pairs of electrons ( π electrons) and a lower reactivity compared with a system containing isolated double bonds. Considering the simplified mechanism of the electrophilic aromatic substitution reaction (SEAr), reported in Scheme 1, the first interaction occurs between the aromatic ring and the electrophilic species affording a positively charged intermediate, named Wheland intermediate ( σ−complex). [3] Scheme 1. General simplified scheme for the electrophilic aromatic substitution reaction. The cationic intermediate (or Wheland) derives from the attack of the electrophilic species to one carbon atom of the aromatic ring with a change of its hybridization from sp 2 to sp 3, as a consequence of the addition to the double bond, and the break of the aromatic conjugated system; the resulting σ−complex, is an high energy intermediate [4] (Figure 1). 1 Figure 1. Energetic levels along the reaction coordinate for the electrophilic aromatic substitution reaction. Finally, the substitution product is obtained by proton loss in the rearomatization step, conventionally considered as the fast and irreversible step of this reaction while the rate- determining step of the overall reaction is considered the formation of the σ intermediate. Based on the above reported, the isolation of the σ−complex is not a very simple goal and it is complicated by the short lifetime of this species and its low concentration during the reaction. [5] Actually the general Scheme of the SEAr depicted in Scheme 1 showing only one intermediate of this reaction, can be considered a simplified Scheme because a lot of investigations carried out principally by J. K. Kochi showed the presence of four steps and three intermediates in the electrophilic aromatic substitution reaction pathway, as reported in Scheme 2.[6-9] Scheme 2 . The general mechanism of the aromatic substitution reaction. The reaction’s pathway reported in Scheme 2 shows that a first step involves a donor- acceptor ( DA ) interaction, in which the electrophile get close to the π−electron cloud of the aromatic system, to obtain a non-covalent complex, usually called π−complex. 2 In a DA complex the electrophile is not localized on a particular atom but is close to the π cloud of the aromatic ring. However some experimental studies, involving electrophiles + + such as Br or NO 2 , showed their preferential localization near to a specific C-C bond before to obtain the final σ− complex. [10] The interaction in the π− complex is weak in nature, and for this reason the activation energy for its formation, is low; this implies that the formation rate for the π− complex is weakly influenced from the substituent groups on the aromatic ring. The identification of some π− complexes has been possible because of their electronic transition in the visible region of the electromagnetic spectra, giving the typical intense color of these complexes; furthermore, under some experimental conditions, these complexes have been crystallized and analyzed by X-Ray diffraction spectroscopy. [5,8,9,11] The next step of the reaction allows the formation of a new σ bond between the two substrates, giving the formation of a covalent complex, the σ-complex. The cyclohexadienic system as the evolution of the π− complex is higher in energy with respect to the starting aromatic compound; this means that the reaction can go in both the directions, depending from the activation energy required to return back to starting materials (loss of the just entered electrophile) or to evolve to substitution product (loss of proton); usually, the proton elimination is favored. Finally, in the third step, immediately after the rearomatization process, the leaving group forms again a π− complex with the aromatic ring before to be finally turned away; a simplified energetic trend for the four steps of the SEAr is reported in Figure 2. Figure 2 . Energetic profile for S EAr. 3 The existence of the Wheland intermediate does not legitimate its direct correlation with the transition state. Dewar was the first to deduct the existence of π− complexes along the reaction’s coordinate and hypothesized that the reaction’s rate could depend also from their stability. [12] In accordance with Hammond’s postulate, assuming that species with similar energies along the reaction’s coordinate will also have similar geometry, it is clear that the transition state higher in energy will be similar to the species with a comparable energy. Thus it is possible to have three different situations: [13] 1. Formation of the π− complex: in this case the transition state higher in energy is similar to the charge-transfer complex ( π− complex). [14] If the formation of the π− complex is the rate-determining step there is no isotopic effect. 2. Formation of the Wheland intermediate: the transition state highest in energy is before the Wheland formation. It has been demonstrated that some reactions exhibit a linear relationship between the rate of substitution and the relative stability of the σ− complex; this observation indicates a correlation between the transition state higher in energy and the Wheland intermediate. 3. Proton elimination: the conventional assumption supposes that the proton departure occurs in a fast step, even if is also possible to observe that the transition state higher in energy precedes the proton elimination. A strong isotopic effect (H/D) is characteristic of this reaction as demonstrated by changing the proton with deuterium; in this case the reaction’s rate changes. On the assumption that the constant for the proton elimination is kH and the one for [2] deuterium is kD, if their ratio kH/kD is high (>2), an isotopic effect is present; in this case the reaction can be affected by basic catalysis phenomena. In conclusion, considering a S EAr, the evaluation of the slow step of the overall reaction is not really simple because a lot of factors can influence the reaction progress as the nature of the electrophiles and of the other substrates in solution and also the effect of different substituents on the aromatic ring that play a fundamental role on the regioselectivity of the reaction. 4 II. NUCLEOPHILIC AROMATIC SUBSTITUTION REACTION (S NAR) Benzene is an electron rich system and this is the reason for its deactivation towards nucleophilic substitution reactions; basically this behaviour depends from the electrostatic repulsion between the π cloud and the nucleophile.
Details
-
File Typepdf
-
Upload Time-
-
Content LanguagesEnglish
-
Upload UserAnonymous/Not logged-in
-
File Pages150 Page
-
File Size-