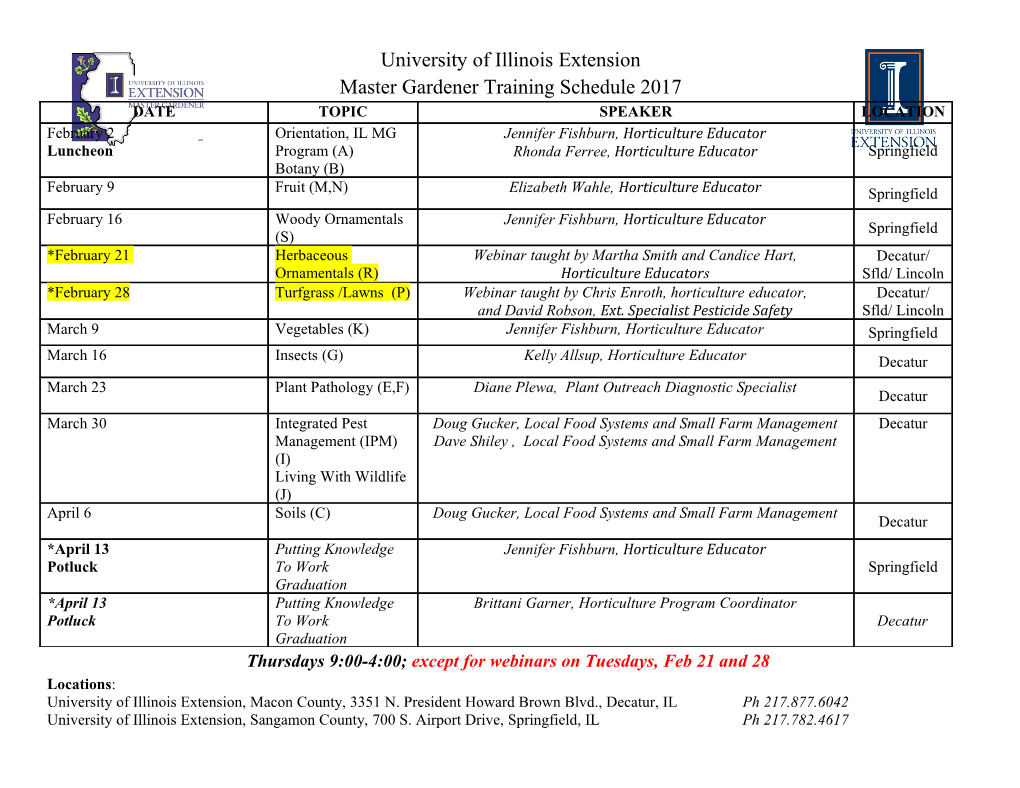
A PULSED HIGH VOLTAGE BULK BREAKDOWN SYSTEM IN VACUUM by WILLIAM FRANKLIN AARON, III, B.S. A THESIS IN PHYSICS Submitted to the Graduate Faculty of Texas Tech University in Partial Fulfillment of the Requirements for the Degree of MASTER OF SCIENCE Approved Accepted May, 1993 ACKNOWLEDGEMENTS I would like to express Slncere gratitude to Dr. Lynn Hatfield for his patience, advice and support throughout my research studies. Also, I am greatly indebted to him for giving me an opportunity to work in his lab and for the many fruitful discussions we had. I would also like to thank Dr. Magne Kristiansen, and Dr. Roger Lichti for being members of my thesis committee. Many thanks are ln order for the Pulsed Power Group and Physics Department staff. In particular, I would like to thank the following people, without whose help this project would not have been completed: Kevin Aaron·, Bob Burch, Paul Grimely, Richard Hernandez, Ellis Loree, Brian McCuistian, Pete Seibt, and Kim Zinsmeyer. Also, I would like to thank Dr. John D. Smith for his technical assistance on the design of the high voltage vacuum bushing. Foremost of all, I wish to give special thanks to my parents, for their encouragement and support throughout the entire graduate program. Finally, I would like to thank SDIO/T/IS through DNA/RAEV for making this work possible. 11 TABLE OF CONTENTS ACKNOWLEDGEMENTS........................................ ll ABSTRACT . v LIST OF TABLES . Vl LIST OF FIGURES . Vll CHAPTER I. INTRODUCTION . 1 II. SURVEY OF BULK BREAKDOWN EXPERIMENTATION . 4 Electrode Geometry 4 Excitation Voltage ........................ 6 Specimen Type ............................. 6 Test Environment .......................... 6 Polarity Effects .......................... 10 Space Charge . 10 Light Emission . 10 III. BULK BREAKDOWN OF INSULATORS . .. .. .. 13 Electron Multiplication Model . 13 Gaseous Channel Model . 15 IV. EXPERIMENTAL SETUP............................. 19 Experimental Chamber . 19 Vacuum System . 21 Marx Generator . 23 DC High Voltage Power Supply............... 32 Triggering Circuit . 32 Inductor . 3 2 High Voltage Vacuum Bushing................. 38 Cathode . 46 Sample Holder . 50 Data Acquisition . 50 V. EXPERIMENTAL RESULTS . .. 58 Marx Generator Results . 58 High Voltage Vacuum Bushing Results . 58 Cathode/Sample Results .. 62 VI . CONCLUSIONS . 6 6 111 ENDNOTES . 6 8 REFERENCES . 72 APPENDICES A. INDUCTIVE CHARGING OF ONE CAPACITOR BY ANOTHER . 76 B. INDUCTANCE CALCULATIONS FOR SINGLE LAYER COILS . 80 C. CAPACITANCE CALCULATIONS ....................... 82 D. FIELD ENHANCEMENT FORMULA DERIVATION . 90 . IV ABSTRACT After 50 years of continuous studies the mechanisms which contribute to the breakdown of insulators through the bulk are still unknown to the engineer or scientist. Many of the experimental setups used in the past employed techniques or equipment which would obscure the precursors which lead to the eventual breakdown. To this end an experimental apparatus has been constructed, in our laboratory, through which the pre-breakdown mechanisms might be discovered. The system consists of a Marx generator, inductor, high voltage vacuum bushing, and a needle-plane electrode geometry. Through the use of the inductor and needle, fields in excess of 1 MV/cm can be obtained. The electrode assembly is situated inside an experimental chamber evacuated to pressures below lxlo-5 Torr. The majority of the thesis is devoted to describing the design and construction of the experimental system. Some time will be spent reviewing the previous methods used to study catastrophic failure along with the theories that have been proposed to explain the phenomenon. Finally, the results of the initial testing and evaluation of the system are presented. It is readily apparent, from the data, that the system will be able to produce the fields necessary to initiate breakdown and to propagate it to a catastrophic failure. v LIST OF TABLES 2.1 Electrical properties for varlous materials. 8 4.1 Experimental measurements of the calibration probe•s attenuation ratio versus period length. 57 5.1 Physical parameters for the testing of the experimental system ......................... 63 5.2 Magnitude of voltage applied to the cathode and the enhanced electric field ............. 65 . VI LIST OF FIGURES 2.1 Arrangement of electrode and sample......... 5 2.2 Tree inception voltage as a function of radius of the needle point.................. 7 2.3 Puncture voltage as a function of radius of the needle point. 7 2.4 Distribution of breakdown fields for (a) unannealed and (b) annealed single crystal specimens of KBr. 9 2.5 Time to breakdown for cross linked and virgin samples. 9 2.6 Effect of mechanical strain on the variation of the time for 50% breakdown probability with voltage ............................... 11 4.1 Pulsed bulk breakdown experimental apparatus 20 4.2 Schematic Vlew of the vacuum system ......... 22 4.3 Schematic of a 4-stage Marx generator ....... 24 4.4 Equivalent circuit of a Marx generator connected to a electrode/sample assembly and the derivation of the equation for the voltage at the sample ....................... 24 4.5 Inductive charging of one capacitor by another . 2 5 4.6 Marx generator employed in the experiment ... 27 4.7 Marx generator with the charging and triggering circuits ......................... 28 4.8 Marx generator and the high voltage interface ................................... 29 4.9 Circuit simulation of the experimental system . 3 1 4.10 The equations used to select the outer radius of the inductor so that the electric field is minimized .......................... 34 4.11 Paschen curve for SF6 in log-log scale ...... 35 Vll 4.12 Maximum field strength, E, with a potential difference, U, between the electrodes for parallel plate geometry and cylindrical geometry. 3 6 4.13 High voltage epoxy feedthrough .............. 39 4.14 Kinetic analysis for Epoxy Technologies epoxy Epo-Tek 301.2 ......................... 40 4.15 High voltage vacuum bushing ................. 42 4.16 Triple point junction detail for the bushing. 4 3 4.17 Oil circulation and conditioning system for the HWB . 4 5 4.18 Equipotential field plot for the cathode with the equipotential lines at 12.5 kV in t e rva 1 s . 4 7 4.19 Equipotential field plot for the needle electrode with the equipotential lines at 12.5 kV intervals ........................... 47 4.20 Schematic representation of the cathode assembly with the accompanying field enhancement equation ........................ 49 4.21 Cathode and the sample holder ............... 51 4 . 2 2 Trigger probe. 52 4.23 Vacuum voltage probe ........................ 54 4.24 Calibration probe ........................... 56 4.25 Calibration curve showing the response of the probe . 57 5.1 Marx generator output under test conditions. 59 5.2 Marx generator output into the HWB, for two shots under identical conditions ............ 61 5.3 High voltage pulses applied to the cathode/ sample geometry. 6 4 Vlll CHAPTER I INTRODUCTION Approximately 50 years ago, scientists and engineers started to report on the mechanism of dielectric breakdown of solid organic insulating materials. During the first fifteen years, considerable effort was directed toward the effects of partial discharges on the surface of the candidate materials with little attention paid to internal degradation leading to breakdown. With the increased demands made on high voltage insulation, bulk breakdown has become a competing or sometimes dominating factor coupled with surface flashover. It has been established in recent years that the breakdown of solid insulation in diverging fields may occur by the action of successive discharges, which cause a tree­ like structure of channels to propagate from the region of high electric stress into the body of the dielectric, until a continuous conducting path lS formed between the electrodes. The time taken for this to occur depends on the circumstances and may vary from many hours to a few seconds. The average speed of the process is, however, not comparable with that of intrinsic electric breakdown, which is about 108 cm/s in a uniform field. The growth of the tree-like structure of channels can be watched and photographed in transparent dielectrics, and Bolton [1] has obtained a film of the breakdown of Perspex, which illustrates the relatively slow step-by-step irregular growth of the discharge channels between point electrodes with 50 Hz alternating voltage. There are two basic types of trees. Trees grown in dry to low humidity environment are referred to as electrical trees, whereas trees grown in a wet environment and usually at lower stresses are known as water, chemical, or electrical trees. Under certain circumstances or conditions, a combination of electrical and electrochemical trees can 1 2 occur. The work described in this thesis will be concerned with the construction of an apparatus to analyze the formation of electrical trees in polymeric materials 1n vacuum which, of course, implies zero relative humidity. Throughout the remainder of this paper, electrical trees will be referred to as trees. The theoretical explanations for bulk breakdown center around two theories: Electron Multiplication and Gaseous Channels. The work of von Rippel and Frolich and their collaborators (reviewed by o•Dwyer [2] and Stratton [3]) between 1931 and 1960 on the electron multiplication mechanism provided what appeared to be a plausible basis for the understanding of the breakdown in solids. However, as new experimental evidence
Details
-
File Typepdf
-
Upload Time-
-
Content LanguagesEnglish
-
Upload UserAnonymous/Not logged-in
-
File Pages100 Page
-
File Size-