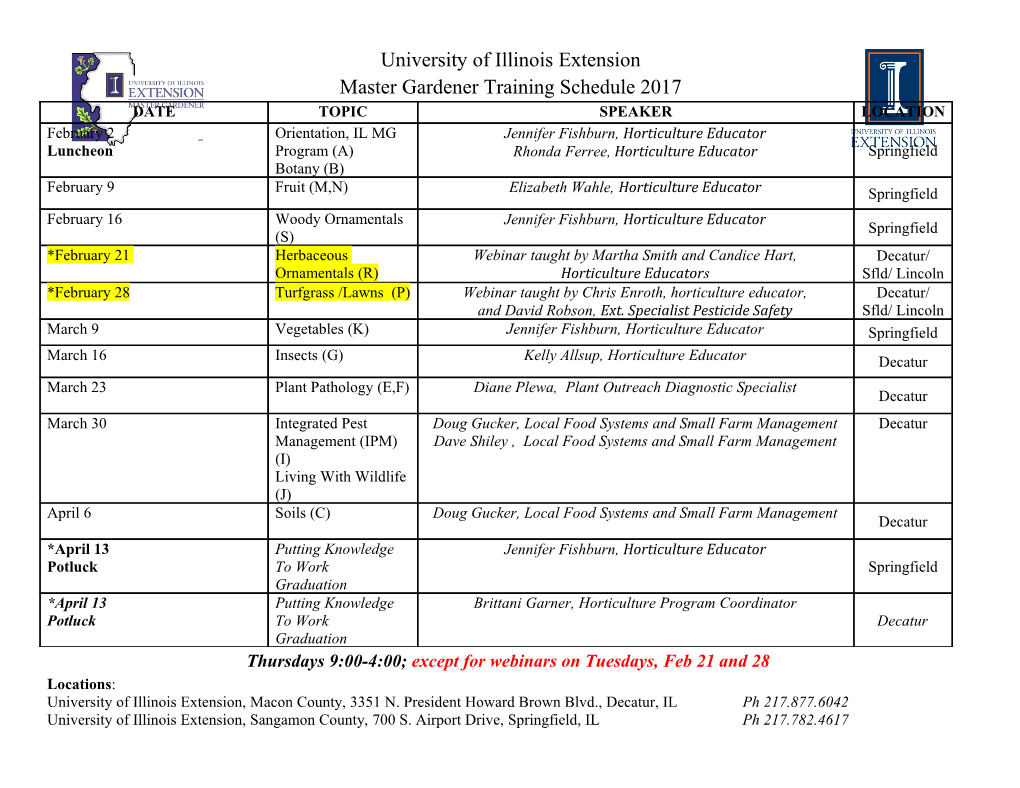
A CHLOROPLAST PHYLOGENY OF AGAVACEAE SUBFAMILY CHLOROGALOIDEAE WITH A FOCUS ON SPECIES RELATIONSHIPS IN HASTINGSIA By KATE MAUREEN HALPIN Master of Science in Botany Oklahoma State University Stillwater, Oklahoma 2011 Submitted to the Faculty of the Graduate College of the Oklahoma State University in partial fulfillment of the requirements for the Degree of MASTER OF SCIENCE May, 2011 A CHLOROPLAST PHYLOGENY OF AGAVACEAE SUBFAMILY CHLOROGALOIDEAE WITH A FOCUS ON SPECIES RELATIONSHIPS IN HASTINGSIA Thesis Approved: Dr. Mark Fishbein Thesis Adviser Dr. Linda Watson Dr. Andrew Doust Dr. Mark E. Payton Dean of the Graduate College ii ACKNOWLEDGMENTS This work would not have been possible without the support of the Oklahoma State University Botany Department faculty and support staff, who I am especially grateful for fully welcoming me into their program during this project’s final year. I would particularly like to thank my thesis advisor Mark Fishbein and committee members Linda Watson and Andrew Doust: their advice and comments improved every stage of this work. Financial support for this work was provided by the American Society of Plant Taxonomists, the Native Plant Society of Oregon, and the Portland State University Creative and Scholarly Activity Grant. iii TABLE OF CONTENTS Chapter Page I. INTRODUCTION......................................................................................................1 Serpentine soils........................................................................................................1 Chlorogaloideae.......................................................................................................5 Species Circumscription in Hastingsia ..................................................................13 Goals ......................................................................................................................16 II. A CHLOROPLAST PHYLOGENY OF CHLOROGALOIDEAE ........................20 Introduction............................................................................................................20 Methods .................................................................................................................22 Results....................................................................................................................27 Discussion..............................................................................................................31 III. A CHLORPLAST PHYLOGENY OF HASTINGSIA .........................................51 Introduction............................................................................................................51 Methods .................................................................................................................54 Results....................................................................................................................59 Discussion..............................................................................................................63 IV. CONCLUSIONS ...................................................................................................80 REFERENCES ............................................................................................................84 iv LIST OF TABLES Table Page 1. Selected characters in Chlorogaloideae and Hesperocallis .....................................19 2. Population locations and vouchers for samples in the Chlorogaloideae study........41 3. Primers used for amplification and sequencing.......................................................43 4. Attributes of the aligned sequences in the Chlorogaloideae study ..........................43 5. Parameter estimates of substitution models in the Chlorogaloideae study..............44 6. Results of topology tests in the Chlorogaloideae study...........................................48 7. Likelihood and posterior probabilities from ancestral character reconstructions....50 8. Population locations and vouchers of Hastingsia....................................................71 9. Attributes of the aligned sequences in the Hastingsia study ...................................75 10. Parameter estimates of substitution models in the Hastingsia study.....................75 11. Results of topology tests in the Hastingsia study..................................................79 LIST OF FIGURES Figure Page 1. Strict consensus of MP trees from the NI dataset for Chlorogaloideae...................45 2. Strict consensus of MP trees from the WI dataset for Chlorogaloideae..................46 3. The ML tree for Chlorogaloideae ............................................................................47 4. Parsimony ancestral state reconstruction of serpentine tolerance ...........................49 5. Likelihood ancestral state reconstruction of serpentine tolerance ...........................50 6. Map of Hastingsia populations in SW Oregon and NW California ........................74 7. Strict consensus of MP trees for Hastingsia............................................................76 8. The ML tree for Hastingsia .....................................................................................77 9. The four TCS haplotype networks...........................................................................78 v CHAPTER I INTRODUCTION SERPENTINE SOILS “Serpentine” is a general term used to encompass all ultramafic rocks and the soils that develop from them, as well as the biotic communities they support. Ultramafic rocks are unique substrates in that their chemical composition is unlike the majority rocks found on the continental crust. Instead, ultramafic rocks are more geologically similar to the sub-oceanic crust from which they originated. At spreading centers in the ocean, liquid magma from the Earth’s mantle seeps out and cools to become new oceanic crust. As an oceanic plate collides with a continental plate, the denser oceanic plate is subducted under and recycled back into the mantle. However, as subduction is occurring, portions of the oceanic plate are accreted onto the continental plate, leaving embedded slabs of ultramafic rocks (Coleman 1977). Over time, suites of plants have become specially adapted to inhabit the distinctive, but harsh conditions found on these serpentine soils developed from ultramafic outcrops. The unique chemistry of serpentine soils creates difficult growing conditions for most plants. Plant growth is limited by high concentrations of iron and magnesium, which are often found at concentrations greater than 70% in the form of ferro-magnesium silicates (Brady et al. 1 2005). Calcium, on the other hand, is limited in serpentine soils, and in the presence of high magnesium concentrations, it becomes even less available to plants (Brady et al. 2005). The low Ca2+/Mg2+ ratios are arguably the biggest influence on plant growth and species composition in serpentine plant communities (Alexander et al. 2007), but there are many other characteristics of serpentine that create edaphic communities. Heavy metals such as chromium, copper, lead, and nickel are found at concentrations toxic to most plant species. Additionally, serpentine soils are generally poor in other essential nutrients (N, K, P), and are often accompanied by erosion and poor water retention. Most plants have adapted over time to the lighter elements that compose the majority of soils developed from the continental crust. However, many plants have adapted to tolerate the heavier elements that make up ultramafics. Plants can tolerate the conditions of serpentine soils in a number of ways. High concentrations of heavy metals can be tolerated by converting the excess metals into non-toxic compounds by the use of chelating agents or by excluding them from entering the plant’s tissues (Gabbrielli et al. 1990; Tilstone and Macnair 1997). Low levels of calcium, on the other hand, are tolerated by either selective uptake of calcium, keeping normal Ca2+ levels within the plant cells, or by tolerating low levels within the plant cells (Marrs and Proctor 1976). However, the adaptive mechanisms that allow plants to survive soils with high concentrations of heavy metals and low Ca2+/Mg2+ ratios are still poorly understood (Brady et al. 2005). Although serpentine soils are most notable for their distinctive chemical composition, they are also accompanied by high levels of erosion and poor water retention (Alexander et al. 2007). Drought tolerance is arguably as important for serpentine inhabitants as the ability to tolerate low Ca2+/Mg2+ ratios and high levels of heavy metals. In a study among serpentine tolerant and serpentine intolerant races of Mimulus guttatus, Hughes et al. (2001) found that drought tolerance was more highly correlated with other habitat characteristics than tolerance of low Ca2+/Mg2+ ratios. Similar studies have shown that drought tolerance was more advantageous 2 than heavy metal tolerance to plants growing on serpentine soils (Freitas and Mooney 1995; Chiarucci 2003). However, not all serpentine sites are dry and barren. Serpentine fens and riparian areas can remain wet and lush throughout the whole year. For example, serpentine fens and seeps support many rare, endemic species that are not found in the drier surrounding chaparrals and savannas, like the carnivore, Darlingtonia californica, or the orchid, Cypripedium californicum. Despite the lush appearance, serpentine fens are still subject to high concentrations of heavy metals, low Ca2+/Mg2+ ratios, and low nutrients,
Details
-
File Typepdf
-
Upload Time-
-
Content LanguagesEnglish
-
Upload UserAnonymous/Not logged-in
-
File Pages102 Page
-
File Size-