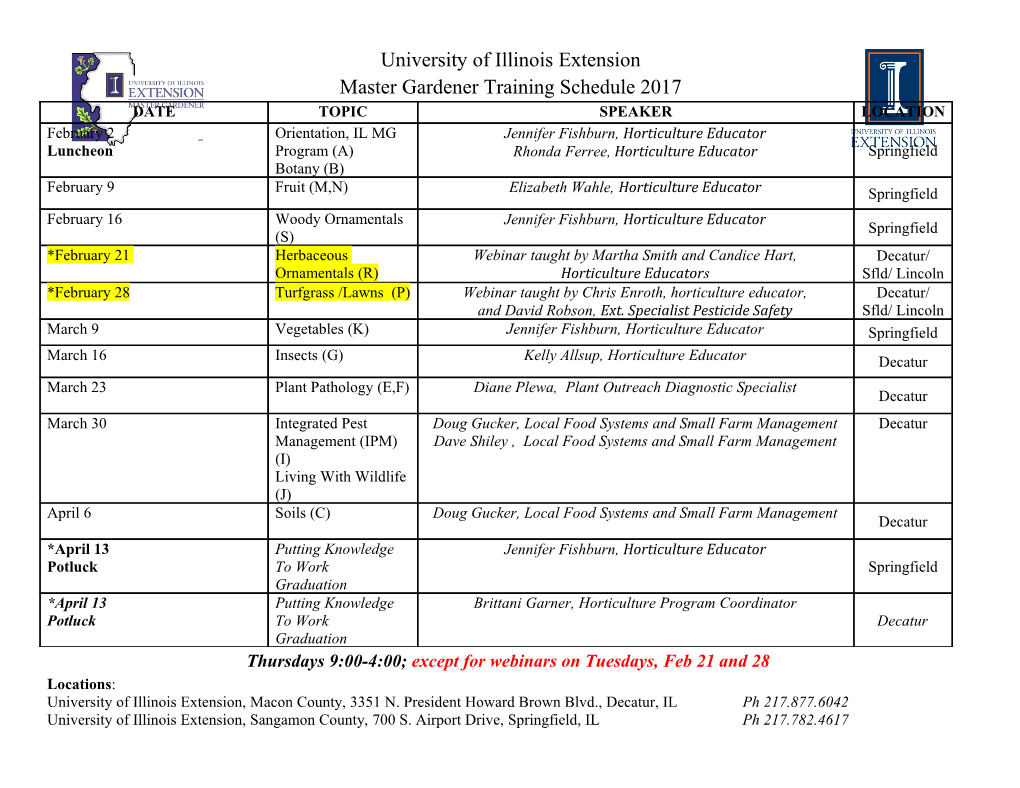
PAPER 5: MOLECULAR ENZYMOLOGY & PROTEIN ENGINEERING Module 17: Enzyme Regulation and Feedback control Introduction: The activity of proteins/enzymes must be regulated so that they function at the proper time and place. The control of enzyme activity is essential in maintaining the steady state of all organisms. The functional expression of particular set of enzymes appears and disappears depending on the cellular need. Therefore, all the biochemical pathways, which can takes place in the cells does not operate in full swing at any given time. Rather, these pathways consisting of enzymatic reactions are regulated in very controlled manner to give optimal function and maintain the homeostasis. For example, glycogen synthesis and breakdown, both the processes occur in liver cells are tightly regulated and work exactly opposite to each other. Depending on the physiological requirement the systemic signals starts glycogen synthesis in liver cells however, when body runs out of energy source, it sends the signal to liver cells for the degradation of stored glycogen. Therefore, both these biochemical processes which acts against each other can takes place in same cells but not at the same time. The disruption of this highly ordered enzymatic control can disturb the homeostasis and can leads to severe pathological conditions. Objectives: In this chapter, we intend to learn the following aspects of Enzyme activity Mechanisms of Control of Enzymatic Activity o Substrate and product concentration o Protein turnover o By expressing various forms of same enzyme o Reversible Post translational Modification o Proteolytic control o Allosteric control Feedback Inhibition Kinetic Behavior of Allosteric Enzymes o Competetive and Non-competitive Inhibition Mechanism of Allosteric Interactions 17.1 Mechanisms of Control of Enzymatic Activity There are variety of mechanisms exist for regulating and controlling the enzyme activity as and when required. The regulation of enzymatic activity and its biochemical reactions starts with synthesis of enzyme (protein) itself. In several proteins/enzymes the activity is regulated by the conversion of enzymes from either “pro” form to “active” form or by posts translational modifications viz. phosphorylation, acetylation etc. On time scale, some of the enzyme activity regulation takes long time; like in cells, the induction of protein synthesis is a complex multistep process that typically requires hours to produce significant changes in overall enzyme level. However, changes in intrinsic catalytic efficiency effected by binding of dissociable ligands (allosteric regulation) or by covalent modification achieve regulation of enzymic activity within seconds. Changes in protein level serve long-term adaptive requirements, whereas changes in catalytic efficiency are best suited for rapid and transient alterations in metabolite flux. The biological activity of Enzymes/proteins is regulated in following ways: 17.1.1 Substrate and Product Concentration: The availability of substrate plays an important role in controlling the enzymatic activity. More concentration of substrate ensures more collision between the substrate and enzyme leading to E+S complex. The rate of reaction of most of the enzymes are responsive to changes in substrate concentration because the intracellular level of many substrates is in the range of the Km. Thus, an increase in substrate concentration prompts an increase in reaction rate, which tends to return the concentration of substrate toward normal. Usually, in any given biochemical pathway consists of many enzymatic reactions in a sequence, only few are controlled/ regulated. For example, the breakdown of glucose in to pyruvate (Glycolysis) occurs in 10 steps, however the first reaction carried out by hexokinase and few more are controlled. They are called rate limiting enzymes. The regulation of rate limiting enzymes occurs in order to conserve energy and metabolites. Sometimes, when the concentration of product is more, the enzymatic reaction gets inhibited and this phenomenon is called feedback inhibition. The first reaction of glycolysis, carried out by hexokinase is very good example of feedback inhibition. Hexokinase phosphorylates glucose to glucose-6-phosphate (G-6-P). If, G- 6-P is not utilized in further consecutive glycolytic reactions, its concentration will increase. Further, it will bind to hexokinase and stops the glucose phosphorylation reaction. This control of enzymatic activity by the end product of the reaction is referred to as negative feedback control. Figure 17a: The diagram depicts, how the substrate and product concentration affects the equilibrium constants Ksyn. And Kdeg., which further controls the enzymatic activity. 17.1.2 Protein Turnover: The first determinant of enzyme activity is the level of enzyme/protein. Enzymes whose concentrations remain essentially constant over time are termed constitutive enzymes viz. metabolic enzyme, Glyceraldehyde-3-phosphate dehydrogenase and structural protein b-Actin. However, the concentrations of many other enzymes depend upon the availability of inducers, substrates or structurally related compounds which initiates their synthesis, for example Escherichia coli grown on glucose will only catabolize lactose after addition of a β-galactoside, an inducer that initiates synthesis of a β-galactosidase and a galactoside permease. Inducible enzymes of humans include tryptophan pyrrolase, threonine dehydrase, tyrosine-α-ketoglutarate aminotransferase, enzymes of the urea cycle, HMG-CoA reductase, and cytochrome P450. On the other hand, an excess of enzyme product synthesis may suppress the enzyme synthesis. Both induction and repression involve cis elements, specific DNA sequences located upstream of regulated genes, and trans-acting regulatory proteins. The absolute quantity of an enzyme reflects the net balance between enzyme synthesis and enzyme degradation (shown in figure 17a). Protein turnover represents the net result of enzyme synthesis and degradation. Enzyme levels in mammalian tissues respond to a wide range of physiologic, hormonal, or dietary factors. For example, glucocorticoids increase the concentration of tyrosine aminotransferase by stimulating the protein synthesis. Regulation of liver arginase can involve changes in protein synthesis and degradation. After a protein-rich meal, liver arginase levels rise and arginine synthesis decreases. Arginase levels also rise in starvation, but here arginase degradation decreases. 17.1.3 By expressing various form of same enzyme: During evolution, developed organisms evolved with various forms of same enzymes for different type of physiological regulation in different tissues. These various forms of enzymes, which catalyze same reaction, are called isozymes. The isozymes provide an avenue for varying regulation of the same reaction at distinct locations or times. Isozymes are homologous enzymes within a single organism that catalyze the same reaction but differ slightly in structure and more obviously in Km and Vmax values, as well as regulatory properties. Often, isozymes are expressed in a distinct tissue or organelle or at a distinct stage of development. For example hexokinase and glucokinase are isozymes found in different tissues. Hexokinase has ubiquitous distribution and has low Km and high affinity for glucose pushes glucose in glycolysis and energy generation (catabolic reaction). However, when systemic glucose exceeds in the blood the glucokinase (found in liver), which has high Km and low affinity for glucose, phosphorylates it to deposit the excess glucose in the form of glycogen (anabolic reaction) in the liver. Figure 17b: Regulation of enzyme activity by phosphorylation dephosphorylation (Covalent modification) at serine, threonine or tyrosine residue. 17.1.4 Reversible Post translational Modifications: The side chain of various amino acids in protein is susceptible to different type of covalent modifications after synthesis. Proteins may be phosphorylated, acetylated, methylated, sulfated, glycosylated, amidated, hydroxylated, prenylated, myristolated, often in a reversible fashion. The catalytic properties of many enzymes are markedly altered by the covalent attachment of a modifying group, most commonly a phosphoryl group on serine, threonine and tyrosine residues. Regulation by phosphorylation through the action of kinases using ATP, and dephosphorylation by phosphates is extremely common (Figure 17b). Control of phosphorylation state is mediated through signal transduction process starting at the cell membrane, leading to the activation or inhibition of protein kinases and phosphatases within the cell. Phosphorylation-dephosphorylation permits the functional properties of the affected enzyme to be altered only for as long as it serves a specific need. Once the need has passed, the enzyme can be converted back to its original form, poised to respond to the next stimulatory event. Another explanation underlying the widespread use of protein phosphorylation-dephosphorylation lies in the chemical properties of the phosphoryl group itself. In order to alter an enzyme’s functional properties (Figure 17c), any modification of its chemical structure must influence the protein’s three- dimensional configuration. The high charge density of protein-bound phosphoryl groups at physiologic pH and their propensity to form salt bridges with arginyl residues make them potent agents for modifying protein structure and function. Phosphorylation generally targets
Details
-
File Typepdf
-
Upload Time-
-
Content LanguagesEnglish
-
Upload UserAnonymous/Not logged-in
-
File Pages12 Page
-
File Size-