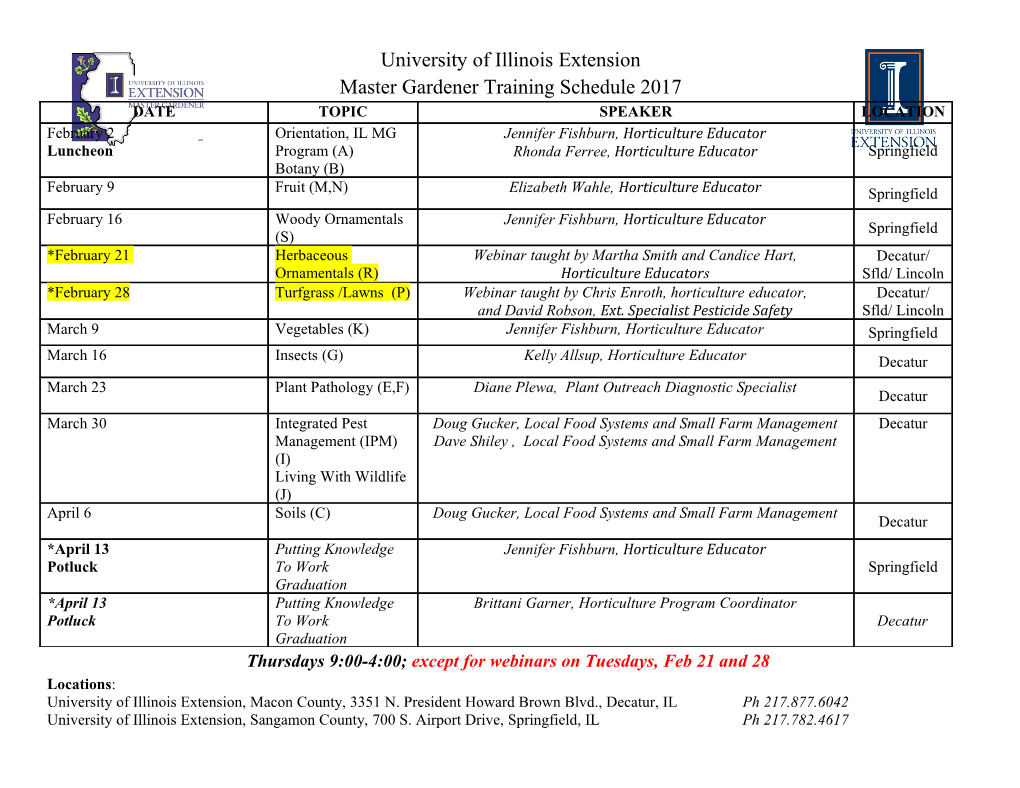
URANIUM CARBIDE AS A NUCLEAR FUEL Uranium carbide has been chosen as the reference fuel material for an organic-cooled reactor study. Acceptable sweiling and fission gas release behaviour have been demonstrated at heat ratings in excess of the design requirements. Where resistance to aqueous corrosion is not re- diation, and is chemically compatible with the quired, as in the organic cooled reactor (OCR), coolant and potential cladding materials under uranium monocarbidc (UC) is an attractive alternative proposed OCR service conditions. Its use in aqueous- (o uranium dioxide (UO2) as a fuel material, because cooled reactors is precluded by its relatively rapid of its 30% higher uranium density and sixfold greater reaction with water. thermal conductivity. These improvements allow The phase diagram of the uranium-carbon system. operation at higher power density, which results in a Figure 1, indicates the existence of three compounds: reduction in reactor core size, and hence a reduction UC, U2C3 and UC2. Sloichiometric UC, which has in capital cost. The use of a high temperature coolant the highest density (13.63 g/cni3) is difficult io such as organic liquid improves the thermal to elec- prepare and impractical on a commercial basis. UC trical conversion efficiency. Uranium carbide has a fuels are classified as hypostoichiometric (<4.80 wt% high melting point (2500°C), exhibits good dimen- carbon) or hyperstoichiometric (>4.80 wt% carbon). sional stability and fission gas retention during irra- The microstructure of hypostoichiometric UC con- 2500 - Fiyure 1 Equilibrium phase diagram of the uranium- p zooq _ carbon system. g 1500 -, 1132 1000 4 5 E 7 CARBON COMPOSITION (wt-%) SO 60 CARBON COMPOSITION tat V.1 13 sists of a fine dispersion of uranium metal within the increase of approximately 2% per 10 MWd/kgU due UC grains or precipitated along the grain boundaries. 10 the accumulation of solid fission products. At Figure 2. As-cast hyperstoichiometric UC consists of higher temperatures increased swelling is attributed to a Widmanstattcn pattern of UC\ platelets on the the precipitation of fission gas bubbles. Initially | lOo} planes of the face-centred cubic UC matrix, formed at high pressure, these swell as a function of Figure 3. temperature and the strength of the fuel. As the UC is isomorphous with the actinide metal mono- temperature increases, gas mobility increases suffi- carbides as well as UN, US and UP. Oxygen also can ciently that after a large amount of swelling has replace carbon in ihc lattice structure up to approxi- occurred gas generation is balanced by gas release and mately 25 atomic per cent, it has been suggested that swelling slows or ceases. oxygen and nitrogon affect the phase equilibria, and Swelling is greater for hypo- than for liyper- possibly the irradiation behavior. stoichiomelric UC. The greater volume increase is UCj has a fee fluorite structure at high tempera- apparently associated with the free uranium metal ture and transforms to a body-centred tetragonal phase. Fission gas diffuses to the uranium at the grain structure at 1820°C. The UC: phase is retained in a boundaries where the fine bubbles nucleate and cause metastable form in material rapidly coole'd from the excessive swelling. In addition the uranium phase melt. It is reported to transform to U1C3 above tends to migrate to the fuel surface leaving porosity I4OO°C under stress or after prolonged annealing in in the central region. vacuum. UjC3 cannot be produced directly by Fission gas release from UC is also greatly depen- casting or compaction of a powder. Once formed at dent on temperature, as with UO->, with indications elevated temperature, it is stable at room tempera- that substantial release occurs above 13OO-I4OO°C. ture. It decomposes to UC and UC2 at approximately Below this temperature gas release is generally <1%, I8OO°C. and below I000°Cit is <0.1% of the amount pro- Various methods have been investigated for Fabri- duced. The quantities released at the lower tempera- cating UC in forms suitable for reactor fuel elements. tures correspond to release by recoil or "knock-out" The most common methods of preparing UC are: by recoiling fission fragments from the fuel surface, and diffusion from surface layers. Gas release from (!) Reduction of UO with carbon, and 2 hypostoichiometric UC is higher than from hyper- (2) carburi/ation of uranium metal with graphite, stoichiometric material. The higher release is caused carbon black or a hydrocarbon gas such as by the presence of free uranium which can provide a methane. network of easy escape paths. The effect is more Fuel rods are fabricated by either melting and casting, pronounced at higher temperatures. Sintered carbide or by powder compaction (sintering, hot pressing, releases more fission gas than cast material, presu- mechanical compaction). These methods produce mably because of the greater surface area. different types of material. Cast material has a larger In contrast to UO-,, structural changes in irra- grain size (> 100 microns), high density (98-99% of diated UC are generally not significant, Figure 4, theoretical) and a low impurity level (<500 ppm since, as a result of its good thermal conductivity, the O+N). Cold-pressed and sintered material has a fuel operates at a smaller fraction of its melting point. smaller grain size 0*10 microns), a iower density Some clacking may occur due to thermal stresses (90-95% of theoretical) and a higher impurity level during power cycling. (1000-5000 ppm O+N). In OCR the fuel is required to operate to a burnup A substantial amount of data has hecn accumu- of roughly 10 MWd/kgU at a linear power output of lated during the past ten years on the irradiation 600 W/cm. The irradiation performance of UC fuel behaviour of UC of various stoichiometries and elements in organic coolant has already been proven methods of fabrication, under a variety of operating to 12 MWd/kgU at <700 W/cm, with sheath tempera- conditions and with a variety of cladding materials tures approaching 500°C. The fast neutron irradiation and heat transfer media. In general the method of facilities in the WR-1 reactor* are fuelled with UC, fabrication appears to be of lesser importance than and prototype 10-cm diameter, 36-element fuel bun- the stoichiometry and density. dles are being irradiated in the U-3 organic-cooled The most important effects of irradiation are loop of the NRU test reactor at outer element linear swelling and fission product gas release, with tempera- power outputs of 650 W/cm to test their suitability ture the most important variable that affects swelling. Below some temperature (=«1100°C) hyperstoichio- *WR-1 is an organic-cooled, materials-test reactor at mctric UC exhibits a minimum isotropic volume the Whiteshell Nuclear Research Establishment. 14 Figure 2 Hypostoichiometric UC (4.53 wt % C) showing presence of free U at grain boundaries (x250). Figure 3 HyperstoichiometricUC(5.07wt % C) showing Widmanstatten pattern of UC: platelets (x250). Figure 4 Hyperstoichiometric UC irra- diated at about 700°C to a burnup of 6.4 MWd/kgU (x250). 15 for an organic-cooled pow?r reactor. Other experi- F.B, Litton, "The Properties and Irradiation Be- ments are in progress in WR-1 to obtain information haviour of Carbide Fuels: A Literature Survey", on swelling and fission gas release at linear power Los Alamos Report LA-3799 (1968). outputs up to 900 W/cm, to burnups of 20 MWd/kgU. B.R.T. Frost, "The Carbides of Uranium", J. Nucl. Mat. 10 (1963), p. 265. R.W. Jones J.A.L. Robertson, "Carbide Fuels and Other Fission- ableCompounds", Chapter 4 in "Irradiation Effects in Nuclear Fuels", Gordon and Breach, For more detailed information see: N.Y. (1969). J.R. MacEwan, et al., "Irradiation Experience With jfc Fuel for Power Reactors", Atomic Energy of Canada Limited Report AECL-3981 (1971). 16.
Details
-
File Typepdf
-
Upload Time-
-
Content LanguagesEnglish
-
Upload UserAnonymous/Not logged-in
-
File Pages4 Page
-
File Size-