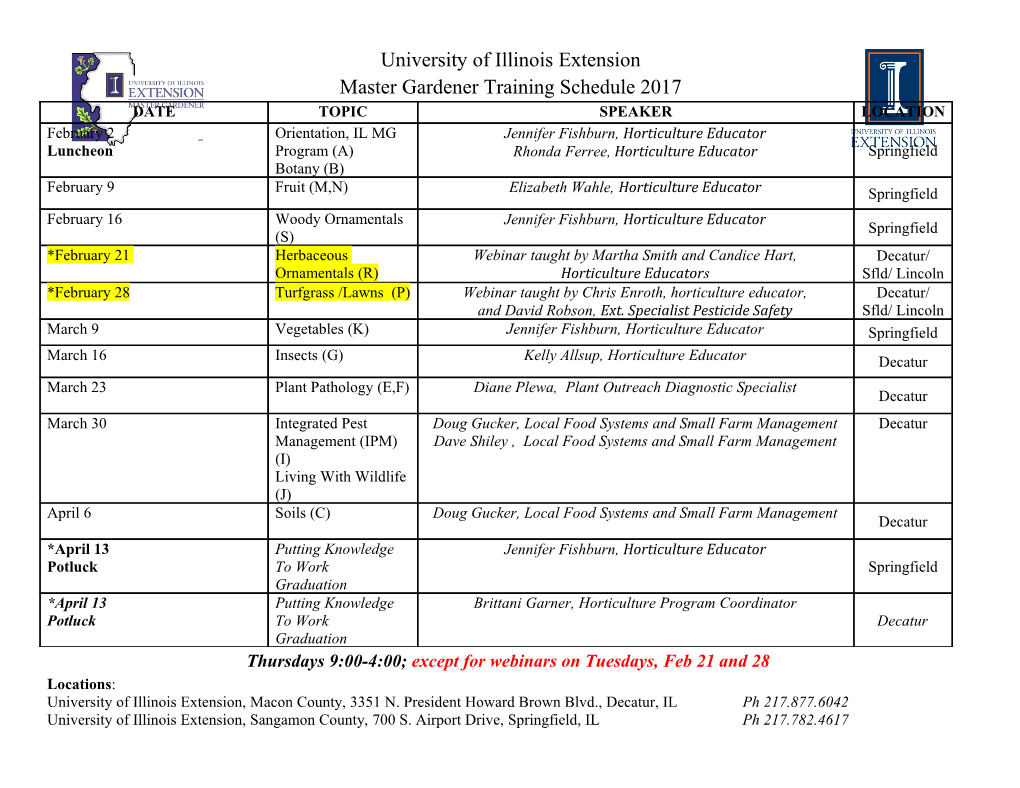
THE RIEMANN HYPOTHESIS IS A TOPOLOGICAL PROPERTY BENOIT CLOITRE Abstract. In this article I present my last updates on a topological approach to the Riemann hypothesis based on the theory of functions of good variation. This is my first attempt to demonstrate the truth of RH. The Riemann hypoth- esis appears to be the consequence of arithmetical, combinatorial, analytical, algebraical and topological phenomena. Foreword Now I (almost) fully agree with the opinion of Ricardo Pérez Marco [Mar]: “Reviewing the analytic progress towards the Riemann Hypothesis is quite frus- trating. The pathetic attempts to enlarge the ridiculous zero free region in the critical strip is a perfect example of what brute force can do without fully exploit- ing fundamental arithmetic aspects of the problem. And we can go on and on, with such examples, where the goal is becoming more and more on improving the epsilons, which evidences the fact that the gap towards the conjectured results will never be filled by such methods without some very original input. Reviewing the algebraic progress towards the Riemann Hypothesis is equally frustrating. Only the toy mod- els for non-transcendental functions have been dealt with. Pushing such methods to transcendental zeta functions has been tried and seems well out of scope. The Grothendieckian approach to divinize and solve with a trivial corollary, does not seem well adapted to the analytic nature of the problem (indeed to any hard con- crete analytic problem). The rich structure of the universe of zeta-functions, allows to combinatorially construct L-functions with a complete lack of analytic informa- tion. This explains the proliferation of interrelated conjectures that after all is just another evidence that some analytic results are missed. One may bet that the real hard problem is the Riemann Hypothesis for Riemann zeta-function, and all the vast other generalizations would fall from the techniques, in a pretty similar way, or with some extra not so hard technique” I say "almost" because the philosophy of Alexander Grothendieck, explained in texts that I have read here and there devoted to his topoï, used as bridges between mathematical theories [Car], have influenced the evolution of my recent thoughts. This led me to a quasi affirmation: not only RH is a topological property but it is a “trivial” corollary of some relation between homeomorphic topological spaces of functions (corollary 68). So I would like to say that the sea has risen around the problem and softened it to the point where it can now be picked like a ripe fruit. That said I won’t use category theory and topoï since my construction in- volves classical general topology (topological spaces, homotopy, homeomorphisms and invariants) which seems sufficient to express my thoughts. Date: Sunday, January 14th 2018. 1 THE RIEMANN HYPOTHESIS IS A TOPOLOGICAL PROPERTY 2 1. Notations and definitions In this section I give notations and definitions which will be commonly used in the paper. Definition 1. Sums If (an)n2 is a sequence and g :]0; 1] ! R is a function then I define N P • A(x) := 1≤k≤x ak P • A1(x) := 1≤k≤x akk P k • Ag(x) := 1≤k≤x akg x Definition 2. Function of good variation (FGV) I say that a bounded function g :]0; 1] ! R satisfying g(1) 6= 0 is a FGV of index α (g) if α (g) is the greatest real value such that for any β < α (g) there exists a non zero constant c(β) such that: −β −β • Ag(n) = n ) A(n) ∼ c (β) n (n ! 1) Definition 3. Dirichlet series and L functions If (an) is a sequence then L(s; a) denotes the Dirichlet series n2N P −s • L(s; a) := n≥1 ann Definition 4. Mellin transform If g :]0; 1] ! R is Riemann integrable I consider the following Mellin transform M[g] which is the meromorphic continuation of the integral defined for <z < 0 by 1 −z−1 •M[g](z) := 0 g(t)t dt and I use the notation´ • g? = M[g] and if G is a space of functions having a Mellin transform then I define ? •M(G) = fg gg2G Definition 5. The analytic index The analytic index of g is defined (when it exists) by • η(g) := min f<(ρ) j ρ 2 C; g?(ρ) = 0g Definition 6. Inverse Mellin transform If g? is a meromorphic function such that there exists g :]0; 1] ! R continuous on ? 1 −z−1 the left such that g (z) = 0 g(t)t dt then g is unique and called the inverse Mellin transform of g? and´ I use the notation • g = M−1[g?] and if G? is a space of functions having an inverse Mellin transform then I define −1 ? •M (G ) = fggg?2G? Definition 7. Broken harmonic functions (BHF) A bounded function g is a BHF if it exists a real positive sequence (rn)n≥1 satis- fying 1 = r1 > r2 > r3 > ::: > r1 = 0 and such that for any n ≥ 1 we have • rn+1 < x ≤ rn ) g(x) = snx THE RIEMANN HYPOTHESIS IS A TOPOLOGICAL PROPERTY 3 where sn > 0 is an increasing sequence of reals such that 8i ≥ 1 we have risi ≤ M for a constant M > 0. Definition 8. Ingham function The Ingham function is defined by 1 • Φ(x) = x x 1 It is a BHF for which ri = i , si = i and M = 1. Here a plot of the Ingham function. Figure 1 Plot of Φ Definition 9. Generalised Ingham functions Let u = (un)n≥1 then the generalised Ingham function associated to u is defined by P 1 • Φu(x) = x 1≤k≤x−1 uk kx It is easy to see that it is a BHF. The Ingham function is obtained with u1 = 1 and un = 0 for n ≥ 2. Here a plot of the generalised function Φχ4 where χ4 = 1; 0; −1; 0; 1; 0; :::: Figure 2 Plot of Φχ4 Definition 10. The Hardy-Littlewood-Ramanujan (HLR) criterion A function g satisfies the HLR criterion (and I say that g is HLR) if for any β ≥ 0 we have the property −β 1−" • Ag(n) = n ) 8" > 0 limn!1 ann = 0 Definition 11. The Riemann functional equation. A function g which is Riemann integrable on ]0; 1] satisfies a Riemann functional equation (and I say that g is RFE) if its Mellin transform satisfies the property THE RIEMANN HYPOTHESIS IS A TOPOLOGICAL PROPERTY 4 g?(z) • <z ≤ 1 ) g?(1−z) 6= 0 I can also say that g? satisfies a Riemann functional equation. Definition 12. Sets and spaces • Z≥m denotes the set of integers greater or equal to m. •F denotes the space of FGV (according to definition 2). •FHLR denotes the subspace of F of functions which are HLR (according to definition 10). •FRFE denotes the subspace of F of functions which are RFE (according to definition 11). •FAff denotes the space of functions defined on ]0,1] which are bounded, affine by parts and continuous on the left. •H denotes the set of arithmetical functions h satisfying the following prop- erties: – h is multiplicative – h(n) n" (Ramanujan condition) P −s – L(s; h) := n≥1 h(n)n converges absolutely for <s > 1 – L(1; h) 6= 0 L(1−z;h) – <z ≤ 1 ) L(z;h) 6= 0 (Riemann functional equation) •LH denotes the set of L functions generated by H i.e. LH = fL(s; h): h 2 Hg 2. Lemmas In this section I state several lemmas. Some proofs are given in the appendices and some of them are classical and are omitted. Lemma 13. The Ingham summation formula n n X jnk X X u = u k k d k=1 k=1 djk Proof. Classical. Lemma 14. Mellin transform of the Ingham function Φ. We have: 1 ζ(1 − z) Φ?(z) := Φ(t)t−z−1dt = ˆ0 1 − z Proof. See APPENDIX 1 Lemma 15. Mellin transform of generalised Ingham functions Φu. We have: 1 ? −z−1 ζ(1 − z)L(1 − z; u) Φu(z) := Φu(t)t dt = ˆ0 1 − z Proof. See APPENDIX 1 Lemma 16. Asymptotic property of a Dirichlet convolution THE RIEMANN HYPOTHESIS IS A TOPOLOGICAL PROPERTY 5 If f is multiplicative and 0 < f(n) ≤ 1 for n ≥ 1 then we have X n µ ? f(n) := µ f(d) = O(1) d djn Proof. See APPENDIX 2. Lemma 17. Two sums involving the divisors If f(n) is defined for n ≥ 1 by n X X j n k f(n) = u(k) v(i) ik k=1 1≤i≤n=k then we have X n X n u (d) v = µ (f(d) − f(d − 1)) d d djn djn Proof. See APPENDIX 2. Lemma 18. Dirichlet inverse of a completely multiplicative function If w is completely multiplicative then w has a Dirichlet inverse given by w−1(n) = µ(n)w(n) . Proof. Classical. Lemma 19. Dirichlet inverse of a multiplicative function If u is multiplicative then the Dirichlet inverse u−1 is also multiplicative. Proof. Classical. Lemma 20. Inverse of multiplicative functions preserve the Ramanujan condition If u is multiplicative and satisfies u(n) = O(n") then its Dirichlet inverse satisfies also u−1(n) = O (n"). Proof. see APPENDIX 2. Lemma 21. The Ingham function is HLR −β −1 More precisely for any β ≥ 0 we have AΦ(n) = n ) an = O(n ). Proof. See APPENDIX 3 Lemma 22. Some generalised Ingham functions are HLR These Ingham functions are HLR X 1 Φ (x) = x u(k) u kx 1≤k≤1=x where u = χ is a Dirichlet character or u is multiplicative and satisfies the Ra- " manujan condition un = O (n ).
Details
-
File Typepdf
-
Upload Time-
-
Content LanguagesEnglish
-
Upload UserAnonymous/Not logged-in
-
File Pages36 Page
-
File Size-