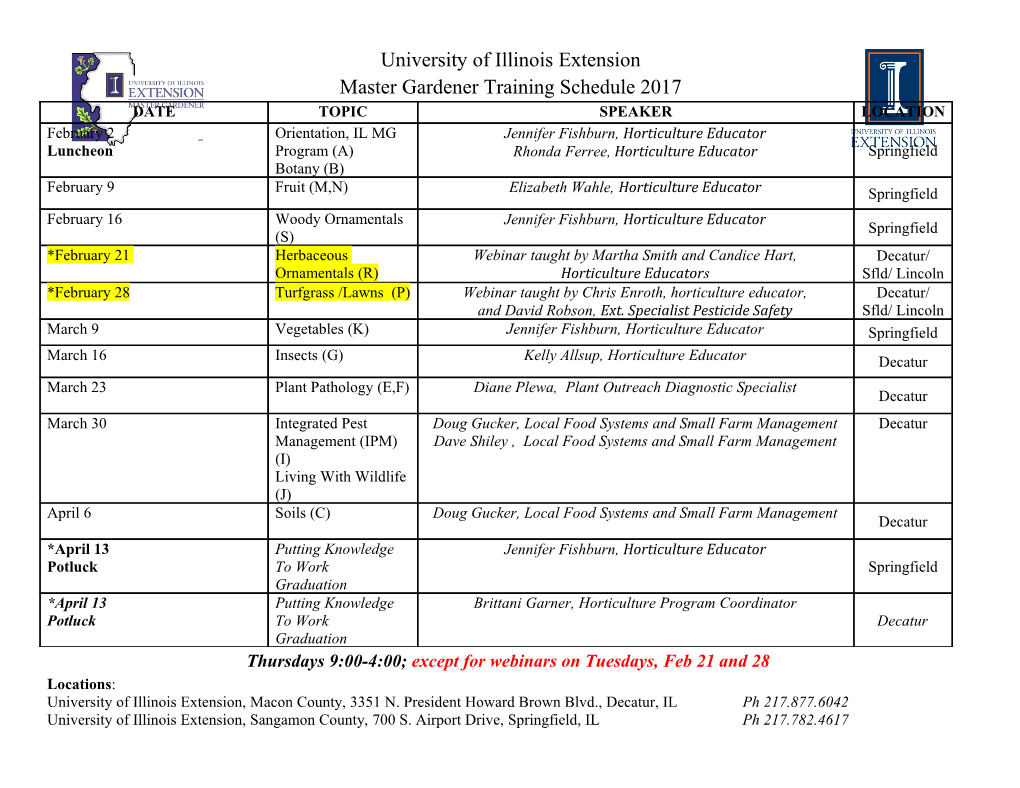
bioRxiv preprint doi: https://doi.org/10.1101/2021.02.19.431970; this version posted February 19, 2021. The copyright holder for this preprint (which was not certified by peer review) is the author/funder. All rights reserved. No reuse allowed without permission. 1 Insights into the regulatory mechanisms of Clostridioides difficile 2 biofilm formation 3 Anthony M. Buckley1, Duncan Ewin1, Ines B. Moura1, Mark H. Wilcox1 & Gillian R. 4 Douce2* 5 1Healthcare Associated Infection Research Group, Molecular Gastroenterology, Institute for 6 Medical Research, University of Leeds, Leeds. U.K. LS1 3EX 7 2Institute of Infection, Immunity and Inflammation, University of Glasgow, Glasgow, U.K. 8 G12 8TA 9 *Corresponding author: Dr Gillian Douce, Email: [email protected], Tel: 10 +44141 3302842, Institute of Infection, Immunity and Inflammation, University of 11 Glasgow, Glasgow, U.K. G12 8TA 12 Running title: C. difficile biofilm formation 13 Key words: Clostridium difficile, biofilm, metabolism, small RNAs 14 1 bioRxiv preprint doi: https://doi.org/10.1101/2021.02.19.431970; this version posted February 19, 2021. The copyright holder for this preprint (which was not certified by peer review) is the author/funder. All rights reserved. No reuse allowed without permission. 1 Abstract 2 Mucosal biofilms play an important role in intestinal health; however, the mucosal bacterial 3 community has been implicated in persistent infections. Clostridioides difficile is an 4 important nosocomial pathogen, with an unacceptable high rate of recurrence following 5 antibiotic treatment. As C. difficile is a known biofilm producer, a property which may 6 contribute to this suboptimal therapeutic response, we have investigated the transcriptional 7 changes and regulatory pathways during the transition from planktonic to biofilm mode of 8 growth. Widespread metabolic reprogramming during biofilm formation was detected, 9 characterised by an increased usage of glycine metabolic pathways to yield key metabolites, 10 which are used for energy production and synthesis of short chain fatty acids. We detected 11 the expression of 107 small non-coding RNAs that appear to, in some part, regulate these 12 pathways; however, 25 of these small RNAs were specifically expressed during biofilm 13 formation, indicating they may play a role in regulating biofilm-specific genes. Similar to 14 Bacillus subtilis, biofilm formation is a multi-regulatory process and SinR negatively 15 regulates biofilm formation independently of other known mechanisms. This comprehensive 16 analysis furthers our understanding of biofilm formation in C. difficile, identifies potential 17 targets for anti-virulence factors, and provides evidence of the link between metabolism and 18 virulence traits. 19 2 bioRxiv preprint doi: https://doi.org/10.1101/2021.02.19.431970; this version posted February 19, 2021. The copyright holder for this preprint (which was not certified by peer review) is the author/funder. All rights reserved. No reuse allowed without permission. 1 INTRODUCTION 2 Clostridium difficile is the most common infective cause of antibiotic associated diarrhoea, 3 causing a huge burden on health care facilities around the world. C. difficile infection (CDI) 4 can manifest as mild, self-limiting diarrhoea to pseudomembranous colitis, colonic 5 perforation and death. The majority of symptoms are due to the production of two large 6 toxins, TcdA and TcdB1, the actions of which cause the loss of epithelial barrier integrity2. 7 The proportion of CDI cases that recur after primary antibiotic therapy is 20-30%3, with most 8 representing relapses due to the original strain. This suggests that C. difficile can occupy a 9 protective niche, which can play a role in long-term persistence/colonisation in vivo. Indeed, 10 we have recently shown that sessile C. difficile cells contribute towards recurrent disease4. 11 The term ‘biofilm’ describes microbial populations enclosed in a self-produced matrix 12 adherent to each other and/or surfaces. Biofilms are ubiquitous in nature and can have 13 substantial benefits/consequences depending on the environment and composition of the 14 biofilm. Within the human gastrointestinal tract (GI tract), there is growing evidence that the 15 microbiota can exist in two forms: luminal planktonic bacteria and mucosal sessile bacteria5, 16 where the formation of biofilms can affect the function of the intestinal microbiome and its 17 interactions with the host6. In healthy individuals, the microbiota exists in a mutualistic state 18 with the host, i.e. the host can use bacterial fermentation products of food as an energy 19 source, and bacteria are provided with favourable conditions for growth. Perturbation of this 20 mutualistic relationship, e.g. following administration of antibiotics, causes profound effects 21 on the status quo of the epithelial layer, the metabolic functions of the gut7 and eliminates the 22 protective colonisation barrier that allows colonisation or outgrowth of potentially pathogenic 23 bacteria, such as C. difficile8. 3 bioRxiv preprint doi: https://doi.org/10.1101/2021.02.19.431970; this version posted February 19, 2021. The copyright holder for this preprint (which was not certified by peer review) is the author/funder. All rights reserved. No reuse allowed without permission. 1 C. difficile can form a mono-species biofilm9 or associate with an existing poly-species 2 biofilm in vitro and in vivo4,10,11. Strong evidence exists to suggest that sessile C. difficile 3 biofilms exist as a heterologous population, made up of vegetative cells, spores and micro- 4 aggregates, all encased in a glycan-rich extracellular matrix, composed mostly of 5 polysaccharide PS-II12,13. Poquet et al.,14 characterised the genetic pathways present in strain 6 630Δerm during mid-stage biofilm development (72 hr growth); sessile cells were found to 7 have a different metabolic profile, remodelled cell wall and envelope and display different 8 cell surface organelles compared with planktonic cells. 9 The genetic factors that control the switch of planktonic motile C. difficile cells to sessile 10 non-motile cells are largely unknown; however, several cell-surface structures, such as Type 11 IV pili (T4P)15,16, flagella17,18 and S-layer12,19 have been shown to play a role in biofilm 12 formation (reviewed in20). Some of these cell surface macromolecules can be genetically 13 controlled by riboswitches in response to the secondary cell messenger Bis-(3’-5’)-cyclic 14 dimeric guanosine monophosphate (c-di-GMP). High c-di-GMP levels increase the 15 expression of the T4P loci21, by interacting with a type II riboswitch (Cdi2_4)22, whilst 16 repressing the expression of the major flagella operon flgB23 via a type I riboswitch 17 (Cdi1_3)22,24. In this way, a single signalling molecule can help coordinate cell lifestyle 18 differentiation within a population. 19 In Bacillus subtilis, there are several genetic pathways that promote biofilm formation, one 20 through post-transcriptional phosphorylation of Spo0A, which in turn regulates the SinI-SinR 21 locus, allowing activation of matrix production genes (reviewed in25). SinR represses the 22 genetic loci needed for biofilm formation, but sinI expression overcomes this repression, 23 leading to biofilm formation. C. difficile genomes contain a two gene locus similar to the 24 genetic arrangement in B. subtilis, corresponding to a homologue of SinR (CD2214 in 630 25 and CDR20291_2121 in R20291). Another gene at this locus, CDR20291_2122, has been 4 bioRxiv preprint doi: https://doi.org/10.1101/2021.02.19.431970; this version posted February 19, 2021. The copyright holder for this preprint (which was not certified by peer review) is the author/funder. All rights reserved. No reuse allowed without permission. 1 reported; however, there is a discrepancy in the literature whether this second gene is a 2 homologue of SinI or if this is an extra SinR homologue (termed SinR’)26–28. C. difficile SinR 3 and SinR’ are master regulators that play a role in regulating biofilm formation, motility, 4 sporulation and toxin production14,27,28. Inhibition of flagella rotation is another genetic 5 pathway that promotes biofilm formation in B. subtilis, through phosphorylation of the 6 pleotropic regulator, DegU29. A similar phenotype has been seen in C. difficile, where 7 inhibition of flagella rotation, either through deletion of flagella motor (motB) or preventing 8 flagella glycosylation, resulted in increased biofilm formation17,30, although the underlying 9 mechanisms for this are unclear. 10 In our study, we define the genetic pathways during early stages of biofilm development in 11 the clinical C. difficile ribotype 027 strain R20291. Through defined chromosomal mutations, 12 we explore the role of CDR20291_2121 during biofilm formation, and the interplay of 13 different regulatory pathways involved biofilm formation. 14 5 bioRxiv preprint doi: https://doi.org/10.1101/2021.02.19.431970; this version posted February 19, 2021. The copyright holder for this preprint (which was not certified by peer review) is the author/funder. All rights reserved. No reuse allowed without permission. 1 MATERIALS AND METHODS 2 Bacterial strains and growth conditions 3 Bacterial strains used in this study are listed in Supplemental Table S1. Escherichia coli 4 TOP10 (Invitrogen, U.K.), used as a cloning host and E. coli CA43431 as a conjugal donor 5 were grown aerobically on Luria Bertani medium supplemented with ampicillin (100 µg/ml-1) 6 or chloramphenicol (15 µg/ml-1) when required. C. difficile strains were routinely grown on 7 either CCEY agar plates supplemented with cefoxitin-cycloserine and 5% horse blood or 8 Brain-heart infusion (BHI) agar in an anaerobic workstation (Don Whitley Scientific Ltd, 9 U.K.) at 37 °C. Brain-heart infusion (BHI) broth supplemented with yeast extract and 10 cycteine was routinely used to grow C. difficile strains. Plasmids used in this study are shown 11 in Supplemental Table S1 and Figure S1. 12 Biofilm growth assay 13 Sterilised 13 mm glass cover slips were placed at the bottom of the well of a 24-well tray.
Details
-
File Typepdf
-
Upload Time-
-
Content LanguagesEnglish
-
Upload UserAnonymous/Not logged-in
-
File Pages36 Page
-
File Size-