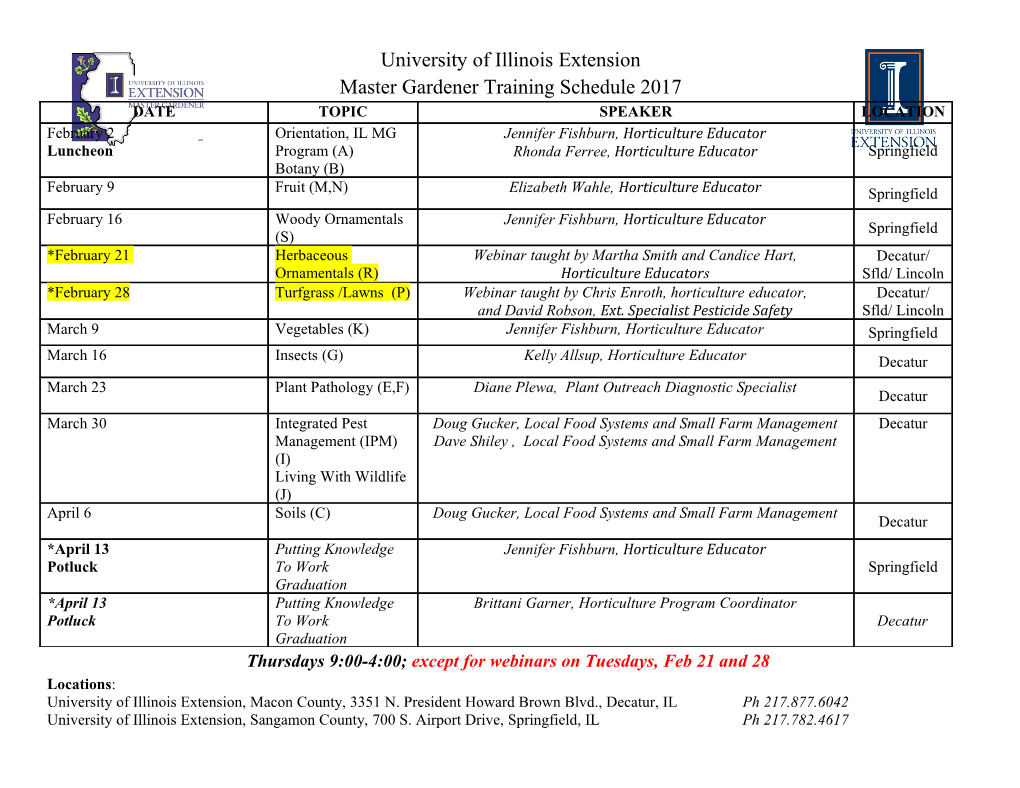
Cationic Photopolymerization of 3- Benzyloxymethyl -3-ethyl-oxetane Kaijing Zheng1, Xiaoqun Zhu1, Xiaochun Qian2, Jun Li2, Chunqing Hu2, Jinliang Yang3*,Rong Bao1 and Jun Nie1* 1 State Key Laboratory of Chemical Resource Engineering, Beijing University of Chemical Technology, Beijing 100029, P.R. China. Fax: (+86) 10-6442-1310; Tel: (+86) 10-6442-1310; E-mail: [email protected]; 2 Changzhou Tronly New Electronic Materials Co., Ltd, Changzhou 213000, China 3 Changzhou Institute of Advanced Materials, Beijing University of Chemical Technology, Changzhou, Jiangsu, 213164, P. R. China. Fax: (+86)519-6969-7567; Tel:(+86)519-6969-7567; E-mail:[email protected] ABSTRACT The cationic photpolymerization of 3-benzyloxymethyl-3-ethyl-oxetane (TR-TCM104) initiated by triphenylsulphonium hexafluoroantimonate (TR-PAG20002) under UV light was conducted. The kinetics was investigated by RT-FTIR and the mechanical and thermal properties of poly(TR-TCM104) were examined by DMA and TGA. To adjust the properties of the polymer, different initiator concentrations, co-monomers were applied. The result showed that the conversion of TR-TCM104 was improved significantly from 17% to almost 90% by adding a certain amount of 3,4-epoxycyclohexane carboxylate or diglycidylether of bisphenol A epoxy resin, while no effects was observed by adding 1,4 - butanediol diglycidyl ether. Moreover, the glass transition temperature, decomposition temperature and Young`s modulus of poly(TR-TCM104) were improved by adding different amount of diglycidylether of bisphenol A epoxy resin. Oxetane conversion and Oxrine conversion at same curing condition has been compared. The physical properties of different cured formulations have been studied. KEYWORDS: Oxetane, Cationic photopolymerization, Diglycidylether of bisphenol A epoxy resin, Copolymerization, Kinetics. INTRODUCTION Over the years, cationic photopolymerization has received great interest as its highlighted advantages over the free radical photopolymerization.1,2 The practical monomers,3-5 susceptible to cationic photopolymerization are less toxic and irritant than other (meth)acrylates6 which are widely used in free radical photopolymerization, are mainly epoxides, vinyl ethers and propenyl ethers.7 According to Crivello,8 the epoxy monomers used in cationic photopolymerization could be divided into three classes. Class I, like cyclohexene oxide has no stable intermediate protonated species (secondary oxonium ions) or tertiary oxonium intermediates, they can polymerize rapidly and exothermically on UV irradiation. Class II, alkyl glycidyl ethers as a representative, they were characterized by an extended induction period because of the stable secondary oxonium ion intermediates. Once initiated, these induction periods are followed by rapid, exothermic autoaccelerated polymerization. Class III, typical monomers 1 like aryl glycidyl ethers, which possess oxygen atoms that are less effective in stabilizing the intermediates and without an obvious induction period but undergo slow, retarded photoinitiated cationic polymerization. Oxetanes as one kind of strained, reactive heterocyclic compounds that undergo cationic ring-opening polymerization were noticed. Their high ring strain energy(107 KJ/mol), is very similar to that of 9 cycloaliphatic epoxides(114 KJ/mol), and the basicity (Pka=2.0) of the heterocyclic oxygen in oxetanes is 10,11 higher than that (Pka=3.7)in epoxides. Moreover, oxetanes could be synthesized by inexpensive, environmentally friendly starting materials12 and have low orders of acute and chronic toxicity and non-mutagenic. Researches about oxetane-based monomers for cationic polymerization have been reported. Yang et al13 fabricated cycloaliphatic epoxy oligo-siloxane (CAEO)/oxetane organic/inorganic hybrid materials which showed a high crosslinking density and flexibility and could be applied to various applications, including optics and display fields. Feser14 reported that oxetane-functionalized organic semiconductors have been used for fabricating multilayer OLEDs from solution and for lithographic patterning. Ghosh et al15 established oxetane-substituted chitosan-polyurethane (OXE-CHI-PUR) crosslinked networks that is capable of self-repairing. Leliège et al16 prepared a photo-patternable electrochromic materials from oxetane precursors. However, it was found that these pure oxetane monomers undergo apparently sluggish reactions and the conversion was very low which makes them generally unsuitable for high-speed automated applications. Earlier work by Wu17 suggested that the rate-determining step for BCOM (3,3-bis(chloromethyl)oxetane) is the ring-opening of the tertiary oxonium ion. In the polymerization of BCMO, monomer was slowly depleted, until after 100 hr the polymerization mixture consisted of 8.2% monomer, 75.4% polymer, and 16.4% tertiary oxonium ion. Spectral Assignments indicated that 40% of the monomer was consumed within the first 5 min. The initial ring opening and formation of tertiary oxonium ions is so fast. After the free acid is consumed, the subsequent ring opening is much slower, and almost 6 hr were necessary for 60% of monomer to be consumed. According to the mechanism commonly accepted of the ring-opening polymerization, the presence of a long-lived tertiary oxonium ion intermediate generated by the reaction of the initially formed secondary oxonium ion intermediate with the cyclic ether monomer limited the initiating rate (Scheme. 1).18 It is assumed that, for this oxetane monomer, the tertiary oxonium ion intermediate is very stable and has very long living time, much more energy is needed to overcome the Ea3 of step 4. Once triggered, the polymerization rate of the oxetane monomers is very rapid and exothermic. The “kick-starting” ring-opening mechanism was also proposed in some of the seminal studies of Crivello.12,19-21 To solve this problem, Crivello18 put forward three general methods for shortening the induction period (1) carrying out the photopolymerization at higher temperature, (2) copolymerizing with more reactive epoxide monomers, and (3) using free-radical photoinitiators as synergists. Under this instruction, Crivello22 studied and found out that by combining with acrylate monomers into hybrid photopolymer systems, 3, 3-disubstituted oxetane monomers can be markedly accelerated. Crivello and Sangermano23,24 prepared the silicon-containing UV curable oxetane monomers and investigated the effects of molecular structures on the curing kinetics and surface properties. The results from photo-DSC showed that the maximum photopolymerization rate decreased while the oxetane conversion increased with the content of reactive polysiloxane increasing. Until now, most of the investigations focused on copolymerization especially with the commercially available cycloaliphatic di-epoxide, 3, 4-epoxycyclohexylmethyl 3, 4-epoxycyclohexanecarboxylate25 and acrylate monomers into hybrid photopolymer. In this work, the method to increase the conversion and the rate of photopolymerization of oxetane monomer (TR-TCM104) was investigated through copolymerizing with less expensive and generally 2 employed commercial available Diglycidylether of Bisphenol A Eoxy resin (DGEBA), and the thermal and mechancal properties of copolymers were examined as well. SCHEME 1 The photoinitiated cationic ring-opening mechanism of TR-TCM104. EXPERIMENTAL Materials Diglycidylether of Bisphenol A Eoxy (DGEBA) was obtained from Jiangsu Sanmu Group Corporation, (Wuxi, China). 3-benzyloxymethyl-3-ethyl-oxetane (TR-TCM104) and triphenylsulphonium hexafluoroantimonate (TR-PAG20002) were both given by Changzhou Tronly New Electronic Materials Co., Ltd. (Changzhou, China). 1, 4-butane dioldiglycidyl ether (BDDGE) was purchased from SY NASIA (Suzhou) Co., Ltd. 3,4-epoxycyclohexane carboxylic acid, methyl ester (EPCM) was purchased from Secco work (Beijing) chemical technology co., LTD. All other agents were purchased from Sinopharm Group Chemical Reagent Co. Ltd. (Beijing, China) and used as received. The chemical structures of the regents were shown in Scheme 2. SCHEME 2 Structures of monomer and photoinitiator. The kinetics of photopolymerization The mixtures of monomer and photoinitiator were applied between two KBr crystals and irradiated by a UV spot light source (EFOS Lite, 100 W miniature arc lamp with 320–500 nm filter and 5 mm crystal optical fiber, Canada) at room temperature. The light intensity was determined by a UV-light Radiometer (Beijing Normal University, China). 3 A real-time IR (RT-IR) spectrometer (Nicolet 5700) equipped with a MCT/A KBr detector-beam splitter combination was used to monitor the photopolymerization kinetics. The spectrometer was operated in the absorbance mode, the polymerization kinetics was determined by the RT-IR spectrometer working in the rapid mode with an average 3 scans s-1 collection rate (4 cm-1) and calculated by the decrease of the area of epoxide group absorption peak around 980cm-1 (oxetane)26 or 915cm-1 (oxirane). The conversion of oxetane (C4%) and oxirane (C3%) were calculated by eq(1) and eq(2), respectively: (퐴960−1006)푡 퐶4% = [1 − ]……eq(1) (퐴960−1006)0 (퐴894−927)푡 퐶3% = [1 − ]……eq(2) (퐴894−927)0 Where (A960-1006)0 and (A960-1006)t were the absorption peak of the oxetane before and after photopolymerization at time t, respectively. (A894-927) 0 and (A894-927) t were for oxirane. The polymerization rate (Rp) could be calculated from the slope of the initial linear portion of the Conversion–Irradiation time curves as follows: 푑(퐶%)
Details
-
File Typepdf
-
Upload Time-
-
Content LanguagesEnglish
-
Upload UserAnonymous/Not logged-in
-
File Pages13 Page
-
File Size-