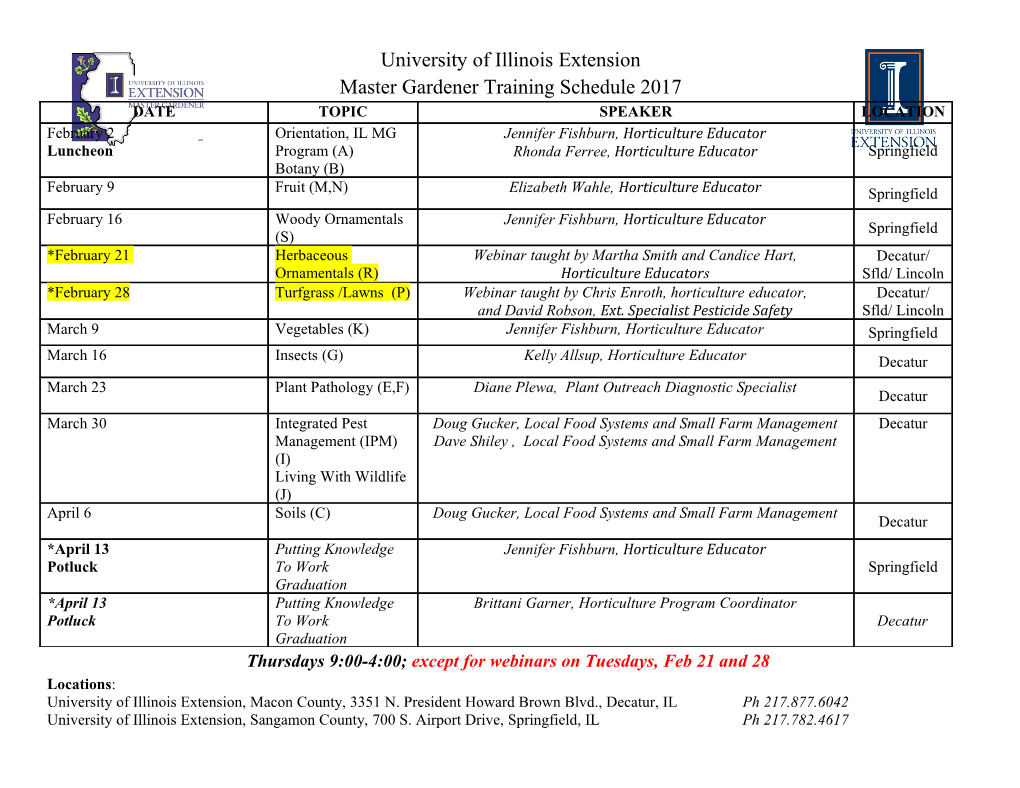
Draft version October 1, 2015 A Preprint typeset using LTEX style emulateapj v. 04/17/13 COMPARATIVE HABITABILITY OF TRANSITING EXOPLANETS Rory Barnes1,2,3, Victoria S. Meadows1,2, Nicole Evans1,2 Draft version October 1, 2015 ABSTRACT Exoplanet habitability is traditionally assessed by comparing a planet’s semi-major axis to the location of its host star’s “habitable zone,” the shell around a star for which Earth-like planets can possess liquid surface water. The Kepler space telescope has discovered numerous planet candidates near the habitable zone, and many more are expected from missions such as K2, TESS and PLATO. These candidates often require significant follow-up observations for validation, so prioritizing planets for habitability from transit data has become an important aspect of the search for life in the universe. We propose a method to compare transiting planets for their potential to support life based on transit data, stellar properties and previously reported limits on planetary emitted flux. For a planet in radiative equilibrium, the emitted flux increases with eccentricity, but decreases with albedo. As these parameters are often unconstrained, there is an “eccentricity-albedo degeneracy” for the habitability of transiting exoplanets. Our method mitigates this degeneracy, includes a penalty for large-radius planets, uses terrestrial mass-radius relationships, and, when available, constraints on eccentricity to compute a number we call the “habitability index for transiting exoplanets” that represents the relative probability that an exoplanet could support liquid surface water. We calculate it for Kepler Objects of Interest and find that planets that receive between 60–90% of the Earth’s incident radiation, assuming circular orbits, are most likely to be habitable. Finally, we make predictions for the upcoming TESS and JWST missions. 1. INTRODUCTION Quintana et al. 2014; Torres et al. 2015; Barclay et al. The discovery of an inhabited exoplanet is a major 2015; Jenkins et al. 2015), Kepler has probably not dis- goal of modern astronomy and astrobiology. To that covered a viable target for transmission spectroscopy. end, NASA and ESA have developed near-term strate- Thus, the K2 and TESS missions are more likely to dis- gies to discover terrestrial planets in the habitable zones cover appropriate targets. As Earth-sized planets have (HZs) of their parent stars with spacecraft such as Ke- transit depths near the detection limit, we should expect pler, K2, TESS and PLATO, and to spectroscopically many false positives, and the full vetting process for these measure the atmospheric properties of transiting exo- candidates could require a massive effort. planets with JWST. Meanwhile, ground-based surveys A transit signal’s planetary origin requires vali- like MEarth could discover 1 additional planet from dation by other means, such as RV measurements the ground (Berta et al. 2013).∼ The Kepler spacecraft (Batalha et al. 2011; Howard et al. 2013; Pepe et al. has discovered a bounty of exoplanets, perhaps over 4000, 2013), photometry by other spacecraft (Ballard et al. orbiting primarily FGK stars (Batalha et al. 2013), al- 2011), adaptive optics (e.g. Kraus et al. 2014), etc. If though only a few are confirmed and potentially habit- we are to achieve the goal of identifying molecular gases able (e.g. Borucki et al. 2013; Torres et al. 2015). These in the transmission spectrum of a potentially habitable results, as well as those from radial velocity (RV) sur- Earth-sized exoplanet, these follow-up observations must veys by the HARPS and HARPS-N instruments (e.g. be made. As these measurements can be expensive, chal- Bonfils et al. 2013), lend confidence to the notion that lenging and time consuming, prioritizing targets can in- TESS will indeed discover tens of potentially habitable crease the probability of success in the search for life in the universe. The goal of this paper is to lay out a sim- arXiv:1509.08922v1 [astro-ph.EP] 29 Sep 2015 super-Earths (1–2 R⊕ and rocky) in the HZs of nearby, bright stars (Ricker et al. 2014; Sullivan et al. 2015) that ple approach to comparative habitability assessments us- will be amenable for JWST follow-up (Deming et al. ing basic transit and stellar data to compare transiting 2009; Misra et al. 2014a; Cowan et al. 2015). planets in terms of their potential to support life. We Absorption features in the transmission spectrum of a define a “potentially habitable” planet to be one that is habitable Earth-like planet are easiest to detect if the mostly rock, with a small (. 100 bar), high molecular host star is apparently bright (Deming et al. 2009). Un- weight atmosphere, and with energy sources and an in- fortunately, the vast majority of Kepler Objects of Inter- ternal structure such that the surface temperature and est (KOIs) orbit faint host stars hundreds of parsecs from pressure permit liquid water for geological timescales. Both K2 and TESS should discover 10 rocky plan- Earth, and despite significant effort to validate poten- ∼ tially habitable planets (e.g. Borucki et al. 2012, 2013; ets in the HZ that could be observed with JWST (Ricker et al. 2014; Beichman et al. 2014; Sullivan et al. 2015). The K2 mission can discover planets in the 1 Astronomy Department, University of Washington, Box HZ of their parent star, but they are likely to be rel- 951580, Seattle, WA 98195 2 atively large and orbiting relatively dim M dwarf stars NASA Astrobiology Institute – Virtual Planetary Labora- tory Lead Team, USA (Beichman et al. 2014). TESS will find smaller planets 3 E-mail: [email protected] around brighter stars and has been designed to search for 2 planets that will be easiest to observe with JWST, see estimate the probability that observational data are con- 4. In both cases (and for Kepler), transit data com- sistent with flux limits from models of habitable planets. bined§ with stellar properties provide relatively limited The value of F depends on L∗, a, the albedo A and information for assessing habitability. We expect transit the eccentricity e: data to accurately constrain the orbital period P , transit L∗(1 A) depth d, and transit duration D, and for the host star’s F = − , (1) 2 2 surface gravity log(g), radius R∗, and effective tempera- 16πa √1 e − ture T∗ to be known. From these 6 parameters we must where we have assumed that the emitted flux is equal prioritize targets for their astrobiological interest. to one-quarter the absorbed flux (the ratio of the cross- In some cases, more information will be available. The sectional area that absorbed the flux to the area of the high cadence TESS data should also provide the impact emitting surface) and have averaged over the orbital pe- parameter b. This addition allows an estimation of the riod (Berger et al. 1993), i.e. the planet quickly advects minimum eccentricity emin of the orbit (Barnes 2007, absorbed radiation to the anti-stellar hemisphere and is 2015). Other transiting exoplanets may also be detected in radiative equilibrium. Note how F depends on e and in some systems, and enforcing stability in those sys- A: Higher eccentricity will lead to higher fluxes, while tems can constrain the maximum eccentricity emax (e.g. higher albedo will lead to lower fluxes. As neither pa- Barnes & Quinn 2001; Shields et al. 2015). When avail- rameter can be well-constrained by transit data, there able these data should also be leveraged in any prioriti- exists an “eccentricity-albedo degeneracy” for potentially zation scheme. habitable transiting exoplanets. We can mitigate this de- Having identified the available parameters, the next generacy by employing constraints on e, but A is usually step is to determine how to prioritize planets in terms unconstrained by the observational data. of habitability. Traditionally, exoplanet habitability is Transit data alone do not provide a constraint on plan- assessed by comparing a planet’s semi-major axis a and etary density ρ, and hence without additional informa- host star luminosity L∗ to the location of the HZ, a shell tion we do not know if an exoplanet is rocky or gaseous. around a star in which an Earth-like planet could sup- As terrestrial planets are the more likely abodes of life, port liquid water (Kasting et al. 1993; Selsis et al. 2007; we will give lower priority to larger planets that are more Kopparapu et al. 2013, 2014). Habitability assessment likely to be “mini-Neptunes,” i.e. small planets domi- using the HZ tends to be binary: Planets in the HZ are nated by gases and ices (Barnes et al. 2009). Some data potentially habitable; those outside it are not. Thus, the and analyses are starting to point to a transition in the HZ itself does not provide the opportunity to determine range of 1.5–2 R⊕ (Marcy et al. 2014; Lopez & Fortney which planets inside are most likely to support life, nor 2014; Weiss & Marcy 2014; Rogers 2015), but the prob- does it explicitly tie transit observables to assessment of ability that a planet is rocky procky cannot be well- a planet’s potential habitability. constrained by transit data alone at this time. To compare planets’ potentials for habitability, one We assume that for a planet to possess surface habit- must quantify the probability that the appropriate con- ability, then it must 1) emit between the limiting fluxes ditions are satisfied. This likelihood is extremely com- of the HZ, and 2) be terrestrial. We then wish to cal- plicated as planetary surfaces evolve chaotically over culate the likelihood that these two conditions are met timescales ranging from seconds to Gyr, and distance based on the available data. We encapsulate this likeli- scales ranging from the atomic to the galactic.
Details
-
File Typepdf
-
Upload Time-
-
Content LanguagesEnglish
-
Upload UserAnonymous/Not logged-in
-
File Pages13 Page
-
File Size-