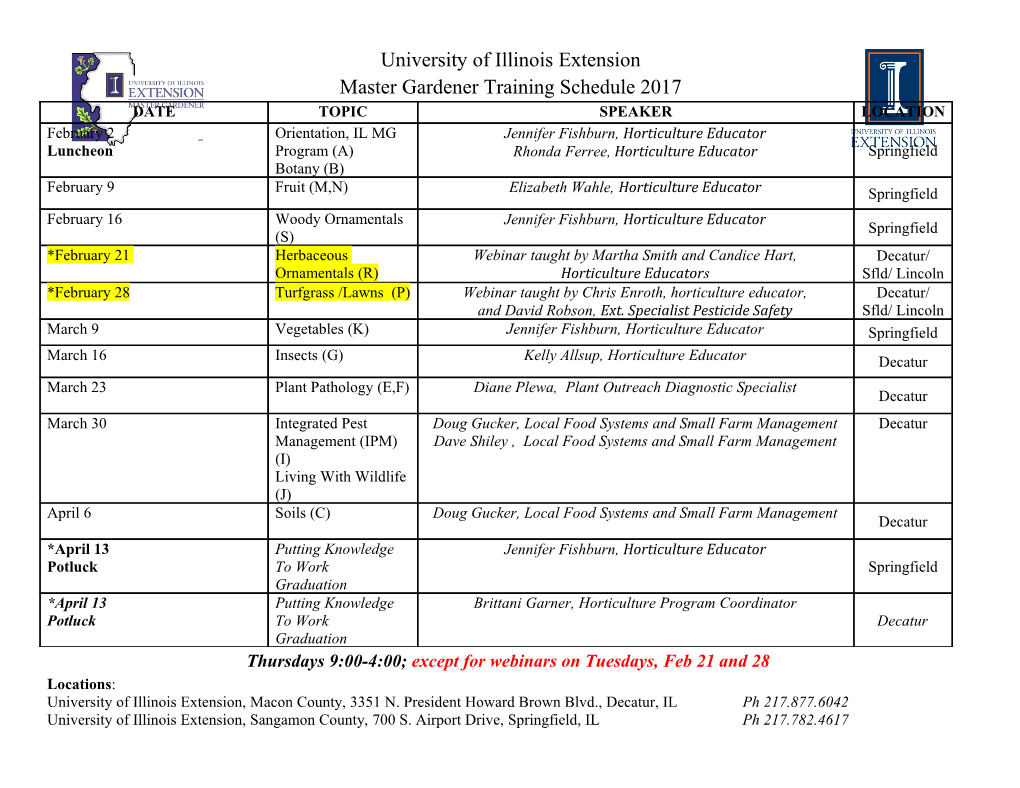
ARTICLES https://doi.org/10.1038/s41561-018-0126-4 Anomalous mantle transition zone beneath the Yellowstone hotspot track Ying Zhou The origin of the Yellowstone and Snake River Plain volcanism has been strongly debated. The mantle plume model successfully explains the age-progressive volcanic track, but a deep plume structure has been absent in seismic imaging. Here I apply dif- fractional tomography to receiver functions recorded at USArray stations to map high-resolution topography of mantle transi- tion-zone discontinuities. The images reveal a trail of anomalies that closely follow the surface hotspot track and correlate well with a seismic wave-speed gap in the subducting Farallon slab. This observation contradicts the plume model, which requires anomalies in the mid mantle to be confined in a narrow region directly beneath the present-day Yellowstone caldera. I propose an alternative interpretation of the Yellowstone volcanism. About 16 million years ago, a section of young slab that had broken off from a subducted spreading centre in the mantle first penetrated the 660 km discontinuity beneath Oregon and Idaho, and pulled down older stagnant slab. Slab tearing occurred along pre-existing fracture zones and propagated northeastward. This reversed-polarity subduction generated passive upwellings from the lower mantle, which ascended through a water-rich mantle transition zone to produce melting and age-progressive volcanism. he mantle plume model for the Yellowstone and Snake River limited to ~300 km in the MTZ and smaller structures may have not Plain volcanism (Fig. 1) has been under much debate for sev- been well resolved. Teleseismic receiver functions, however, can be Teral decades1–4. Seismically slow (hot) anomalies in the upper- used to provide complementary constraints with better lateral reso- most ~200 km of the mantle beneath Yellowstone and the Snake lution at depths of the MTZ as they utilize waves converted directly River Plain have been consistently imaged in recent tomographic at seismic discontinuities near receivers. However, to date receiver studies in the western United States, but a continuous hot plume function studies have been unable to resolve the disparity of opinion structure down to the deep mantle has been absent5–9. The most about the plume origin of the Yellowstone volcanism largely due to dominant seismic features in the mid mantle are fast (cold) anoma- the limitations of back-projection imaging methods used in those lies associated with the subducted oceanic Farallon Plate, currently studies14–16. in the North American mantle transition zone (MTZ)6–12. The geometry of the fast anomalies indicates a strong slab fragmentation Finite-frequency receiver function tomography that created ‘gaps’ in an otherwise spatially continuous feature in The mantle transition-zone discontinuities, that is, the 410 km dis- tomographic images8–12. The absence of a continuous mantle plume continuity and the 660 km discontinuity, are associated with pres- down to the lower mantle in seismic images has been attributed to sure-induced olivine phase transformations. The actual depths at possible complex interactions between a narrow uprising plume which these phase transitions occur depend strongly on the temper- beneath the Yellowstone and the massive downgoing Farallon slab ature and water content17,18. In the classic plume model, the 660 km in the North American mantle3,7–10. However, geodynamical simula- discontinuity is expected to occur at a depth shallower than 660 km, tions have been unsuccessful in reproducing the age migration of the whereas the 410 km discontinuity occurs at a depth greater than volcanic track at Yellowstone in a complex subduction environment 410 km. The depths of the two discontinuities can be constrained when seismic constraints are applied4,13. This controversy left sub- using receiver functions constructed from horizontal- and vertical- duction-driven mantle flows as alternative models for Yellowstone component P-wave coda seismograms because teleseismic P waves volcanism, for example, the eastward intrusion of hot Pacific mantle generate secondary converted S waves at the seismic discontinuities driven by the subduction of the Farallon Plate13 or possible upwell- that arrive later in the P-wave coda. Traditional receiver function ings around a fragmented slab during rapid trench retreat9. imaging is based on back-projection using ray theory, which breaks A major ‘slab gap’ in seismic wave speed was first published in down for structures that are comparable to or smaller than the size 20087 and later confirmed by other seismic studies based on data of the Fresnel zone19. To obtain high-resolution depth variations recorded at the USArray seismic network, which has provided of the two discontinuities in the western United States, I measured unprecedented data coverage in the United States with an aver- finite-frequency travel times of P410 and P660 receiver functions age station spacing of about 70 km. The slab gap is most evident recorded at USArray Transportable Array (TA) stations and imaged in the MTZ in which subduction becomes stagnant just above the the topography of the two discontinuities based on a newly devel- 660 km discontinuity and extends northeastward from the Oregon oped finite-frequency tomographic theory for receiver functions19. and Idaho border, roughly following the Yellowstone volcanic track. Finite-frequency imaging theory is more general than ray theory The exact cause of the slab gap was unclear, but different subduc- as it accounts for wave diffractional effects and, therefore, can be tion angles have been suggested as a possible mechanism7,10. The applied to recover small-scale slab structures that are often in the size and location of the slab gap vary among different wave-speed order of a couple of hundred kilometres20, which may not be resolv- studies because spatial resolutions in these models are generally able in traditional receiver function analysis19. Department of Geosciences, Virginia Tech, Blacksburg, VA, USA. e-mail: [email protected] NATURE GEOSCIENCE | www.nature.com/naturegeoscience © 2018 Macmillan Publishers Limited, part of Springer Nature. All rights reserved. ARTICLES NATURE GEOSCIENCE 48° N 46° N 2.0 44° N 6.6 10.2 42° N 10.5 12.7 16.5 15.9 MFZ 40° N PFZ 38° N 36° N 128° W 124° W 120° W 116° W 112° W 108° W Fig. 1 | Location of the Yellowstone hotspot track. Triangles indicate general locations of the Yellowstone and Snake River Plain age-progressive volcanoes (age in millions of years), plotted on a topography map of the western United States. MFZ, Mendocino Fracture Zone; PFZ, Pioneer Fracture Zone. The dark lines indicate major geological boundaries. The most striking feature in the high-resolution 410 km and this track is shallower than that in the surrounding mantle in which 660 km discontinuity topography maps is a trail of anomalies the associated olivine phase transformation occurs deeper than in beneath Yellowstone and the Snake River Plain, which extends in a normal mantle, as expected in a region with a large stagnant slab the northeast–southwest (NE–SW) direction and closely follows near the 660 km discontinuity. If we assume that seismic anomalies the surface volcanic track (Fig. 2). The 660 km discontinuity along in the lower MTZ are caused dominantly by temperature variations, a b 48° N 48° N 46° N 46° N 44° N 44° N 42° N 42° N 40° N 40° N 38° N 38° N 36° N 36° N 125° W 120° W 115° W 110° W 105° W 100° W 125° W 120° W 115° W 110° W 105° W 100° W –20 0 20 –30 0 30 Depth (km) Depth (km) c d 48° N 48° N 46° N 46° N 44° N 44° N 42° N 42° N 40° N 40° N 38° N 38° N 36° N 36° N 125° W 120° W 115° W 110° W 105° W 100° W 125° W 120° W 115° W 110° W 105° W 100° W –30 0 30 –20 0 20 MTZ thickness (km) MTZ depth perturbation sum (km) Fig. 2 | Mantle transition-zone discontinuities from receiver function diffraction tomography. a,b, Depth perturbations of the 660 km (a) and 410 km (b) discontinuities, respectively. c, Thicker MTZ where the two discontinuities are anticorrelated and perturbations are larger than uncertainties (3 km for the 660 km and 5 km for the 410 km discontinuities). The anomalies can be explained by a possible vertical slab throughout the MTZ, except for the anomaly at Yellowstone where the thicker MTZ corresponds to the trench of the reversed polarity subduction (Fig. 5). d, The sum of discontinuity-depth perturbations where both discontinuities are deeper, which is indicative of possible vertical upwellings through a hydrated MTZ. NATURE GEOSCIENCE | www.nature.com/naturegeoscience © 2018 Macmillan Publishers Limited, part of Springer Nature. All rights reserved. NATURE GEOSCIENCE ARTICLES a b MFZ MFZ c d Normal subduction Fig. 3 | 3D rendering and re-examination of S-wave slab anomalies in the western United States. The isosurface represents 1% fast seismic wave-speed anomalies at depths from 50 km to 1,600 km (ref. 10). US Reversed subduction state boundaries are plotted at the surface for geographical reference. Fast anomalies in the upper mantle beneath the craton are removed for a Fig. 4 | Cartoon illustration of the stages of subduction in the western better illustration of the slab. Map views of the same wave-speed model at United States (not to scale). a, Stagnant slab subduction > 30 Ma. b, Ridge different depths are plotted in Supplementary Fig. 8. The horizontal plane in subduction and slab break off ~25 Ma. c, Reversed subduction (polarity grey is plotted at a 660 km depth for reference. A cartoon representation of reversal) ~16 Ma. d, Present-day slab geometry as seen in Fig. 3: slab tearing this figure is plotted in Fig.
Details
-
File Typepdf
-
Upload Time-
-
Content LanguagesEnglish
-
Upload UserAnonymous/Not logged-in
-
File Pages6 Page
-
File Size-