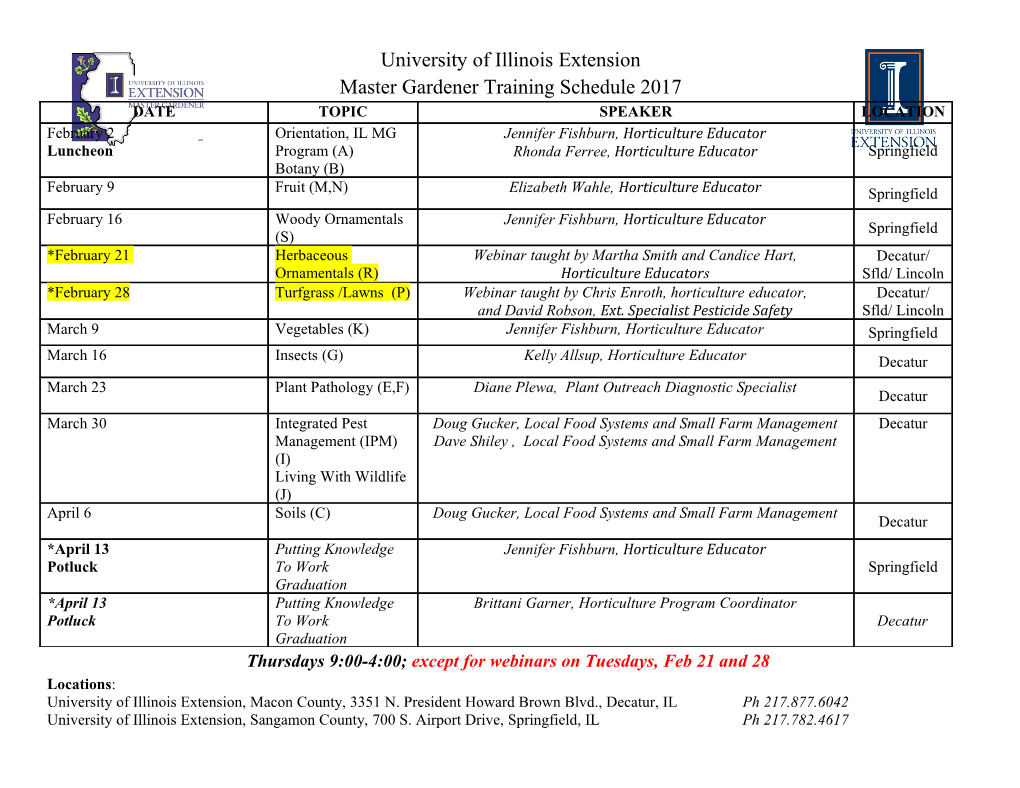
Optimization of a 50 MHz Frequency Modulated Continuous Wave Radar System for the study of auroral E-region coherent backscatter A Thesis Submitted to the College of Graduate Studies and Research in Partial Fulfillment of the Requirements for the degree of Master of Science in the Department of Physics and Engineering Physics University of Saskatchewan Saskatoon By Gareth William Perry ⃝c Gareth William Perry, July 2010. All rights reserved. Permission to Use In presenting this thesis in partial fulfilment of the requirements for a Postgrad- uate degree from the University of Saskatchewan, I agree that the Libraries of this University may make it freely available for inspection. I further agree that permission for copying of this thesis in any manner, in whole or in part, for scholarly purposes may be granted by the professor or professors who supervised my thesis work or, in their absence, by the Head of the Department or the Dean of the College in which my thesis work was done. It is understood that any copying or publication or use of this thesis or parts thereof for financial gain shall not be allowed without my written permission. It is also understood that due recognition shall be given to me and to the University of Saskatchewan in any scholarly use which may be made of any material in my thesis. Requests for permission to copy or to make other use of material in this thesis in whole or part should be addressed to: Head of the Department of Physics and Engineering Physics 116 Science Place University of Saskatchewan Saskatoon, Saskatchewan Canada S7N 5E2 i Abstract A 50 MHz Frequency Modulated Continuous Wave (FMCW) radar system, de- veloped at the University of Saskatchewan to provide improved spatial and temporal resolution measurements of auroral E-region plasma processes, introduces ambiguous spectral information, due to spectral ghosting, for scattering events in which multiple radar echoes are detected. This thesis identifies two Linearly Frequency Modulated (LFM) radar waveforms used by the FMCW system as the source of the ghosting. An analysis procedure designed to counteract the spectral ghosting problem is devel- oped but is not an ideal solution, and therefore replacement of the LFM waveforms is recommended. A detailed investigation of alternative radar waveforms using the Ambiguity Func- tion and Ambiguity Diagram techniques is performed. A frequency coded continuous wave radar waveform based on a composite Costas sequence is proposed as a suc- cessor to the LFM waveforms. The composite Costas radar waveform will conserve the spatial and temporal resolutions extended by the LFM waveforms and preclude any spectral ghosting. Implementing the proposed radar waveform and avoiding re- ceiver saturation issues with the mono-static FMCW radar system in which both the transmitting and receiving antenna arrays are simultaneously and continuously active and geographically co-located is also discussed. In addition to this, two 50 MHz backscatter events are presented in this thesis to demonstrate the effectiveness of the FMCW system, notwithstanding the spectral ghosting complication. The first event from November 21, 2009 is identified as a Type 1 instability and the second from September 13, 2009 is identified as a Type 2 instability which lasted for ∼ 16 minutes. Linear plasma fluid theory is used to provide a brief interpretation of both scattering events. ii Acknowledgements I would like to thank my supervisor Dr. Glenn Hussey for providing me with the opportunity to work on this project. His insight, patience and guidance are truly ap- preciated. I would also like to acknowledge the members of my advisory committee: Dr. Tom Steele, Dr. Kathryn McWilliams, Dr. Adam Bourassa and Dr. Eric Salt. I am grateful for the financial support received from the Natural Sciences and Engi- neering Research Council of Canada (NSERC), the Canadian Space Agency (CSA) and both the Department of Physics and Engineering Physics and the Institute of Space and Atmospheric Studies (ISAS) at the University of Saskatchewan. Special thanks to Jan Wiid for his efforts with the FMCW system. Further thanks to Bill Marshall, Brad Krug, Elise Normand, Marian Thorpe, Jeremy Tran and Joel Cooper for all of their help. Thanks to Dennis and Solange Bakker for their regular site check-ups. Also, thanks to whoever shot three bullets through the FMCW radar shed. You added an edge of danger to my project. Dr. George Sofko deserves thanks for his enlightening conversations, Dr. Jean- Pierre St. Maurice for his candor and to Dr. Pasha Ponomarenko, Dr. Chad Bryant and Dr. Dieter Andr´efor their useful discussions. I thank my fellow students, both graduate and undergraduate including Rob and Megan Gillies for their camaraderie as well as John McLeod and Robin Kleiv for their genuine friendship. I would also like to recognize Dr. Derek Harnett for encouraging me to pursue graduate studies and the staff at the University of the Fraser Valley, W.J. Mouat Secondary School and Centennial Park Elementary school in Abbotsford, B.C. for their commitment and hard-work. To all of my friends in British Columbia and my extended family in England and Canada, I thank you for your love and confidence. To Mom, Dad, Michael and Quinn. Words cannot describe your love, efforts and patience. To Janelle, my wind, my fire, my sunrise and my sunset. iii For Mom, Dad, Michael and Quinn with love. iv Contents Permission to Use i Abstract ii Acknowledgements iii Contents v List of Tables vii List of Figures viii List of Abbreviations xiii 1 Introduction 1 1.1 The Heliosphere . 1 1.2 The Magnetosphere . 2 1.3 The Ionosphere . 2 1.3.1 E region . 3 1.4 Coherent Backscatter Radar . 5 1.4.1 Radar Types . 6 1.4.2 FMCW Radar System . 7 1.5 Thesis Layout . 10 2 Radar Signals and the FMCW Radar 12 2.1 Matched Filter . 12 2.2 Ambiguity Function . 16 2.2.1 Ambiguity Function Properties . 17 2.2.2 Graphical Representations . 20 2.2.3 Linear Frequency Modulated Waveform . 27 2.3 The FMCW Radar System . 32 2.3.1 Data Association . 37 2.4 Other Radar Systems . 42 2.4.1 SuperDARN Radar System . 43 2.4.2 Manastash Ridge Radar System . 45 3 Analysis Technique Modifications for the FMCW System 48 3.1 Two-Bandwidth Sweep Solution . 48 3.1.1 Two-BW Sweep Performance . 50 3.2 Peak-Finder Solution . 58 v 3.2.1 Spectral Peak Identification . 59 3.2.2 Noise Associated Peak Reduction . 60 3.2.3 Peak-Finder Algorithm . 61 3.3 Refined SNRA Calculation . 65 4 Waveform Solutions 68 4.1 Continuous Wave and Pulsed Radars . 68 4.2 Pulse Waveform Parameters . 70 4.2.1 Pulse Duration . 71 4.2.2 Waveform Duration . 72 4.2.3 Pulse Repetition Frequency . 72 4.2.4 Uniqueness Duration . 74 4.3 Unique Pulse Radar Waveforms . 75 4.3.1 PRF Staggering . 77 4.3.2 Phase Coding . 79 4.3.3 Frequency Coding . 82 4.4 Costas Waveforms . 86 4.4.1 Composite Costas Waveforms . 91 4.5 Hardware Parameters . 99 4.5.1 Signal Generation . 99 4.5.2 Bandwidth . 104 4.5.3 Radar Array Configuration . 107 4.5.4 Feed-through . 107 4.5.5 Continuously Active Radar System . 110 4.5.6 Data Association Solution . 113 5 Data and Observations 116 5.1 E-region Plasma Instabilities . 116 5.1.1 Auroral E-region Plasma Theory . 118 5.2 Type 1 Radar Echo . 122 5.3 Type 2 Radar Echo . 125 5.3.1 Type 2 Echo Event . 127 6 Summary and Conclusions 135 6.1 Future Development | Radar Hardware . 137 6.2 Future Development | Radar System . 138 A Costas Arrays 145 B AWG5000B Series Data Sheet 148 vi List of Tables 1.1 Table of parameters for the FMCW radar. This table is a modified version of one by Cooper [2006]. 8 2.1 A comparison of the FMCW, MRR, SuperDARN and STARE radar systems. This table is a modified version of one by Lind [1999]. The velocity information of the SuperDARN system is derived from a fit- ting process and thus does not have a resolution as defined in this section. The MRR system is CW and therefore has an infinite wave- form duration, T .............................. 43 4.1 A summary of the FMCW radar range and frequency resolution along with the range and frequency alias properties and the parameters of the FMCW waveform and the candidate Composite Costas Waveform presented in Section 4.4.1. The properties of a traditional pulse radar waveform used by the Valensole HF radar system is shown as well [Six et al., 1996]. 71 4.2 A summary of the χ% values, the ACF side-lobe suppression val- ues, the range resolution ∆R and frequency resolution ∆f for the radar waveforms discussed in this thesis. In all pulsed waveform cases tb = 20 µs was used and for the LFM case B = 50,000 Hz was used. The χ%, ∆R and ∆f values for a Dirac delta spike is also provided. 98 A.1 Number of Costas arrays [Levanon and Mozeson, 2004a; Drakakis et al., 2008; Rickard et al., 2006]. 146 vii List of Figures 1.1 FMCW radar field-of-view with magnetic contours of 60◦, 65◦ and 70◦. 9 2.1 The amplitude (top), phase (middle) and frequency (bottom) as a function of t=T for a single constant amplitude pulse with a constant carrier frequency. Here, t is time and T is the waveform duration, which is equivalent to the pulse duration. 21 2.2 The AD for a constant amplitude and frequency square pulse. The bottom plot is an overhead view of the AD.
Details
-
File Typepdf
-
Upload Time-
-
Content LanguagesEnglish
-
Upload UserAnonymous/Not logged-in
-
File Pages174 Page
-
File Size-