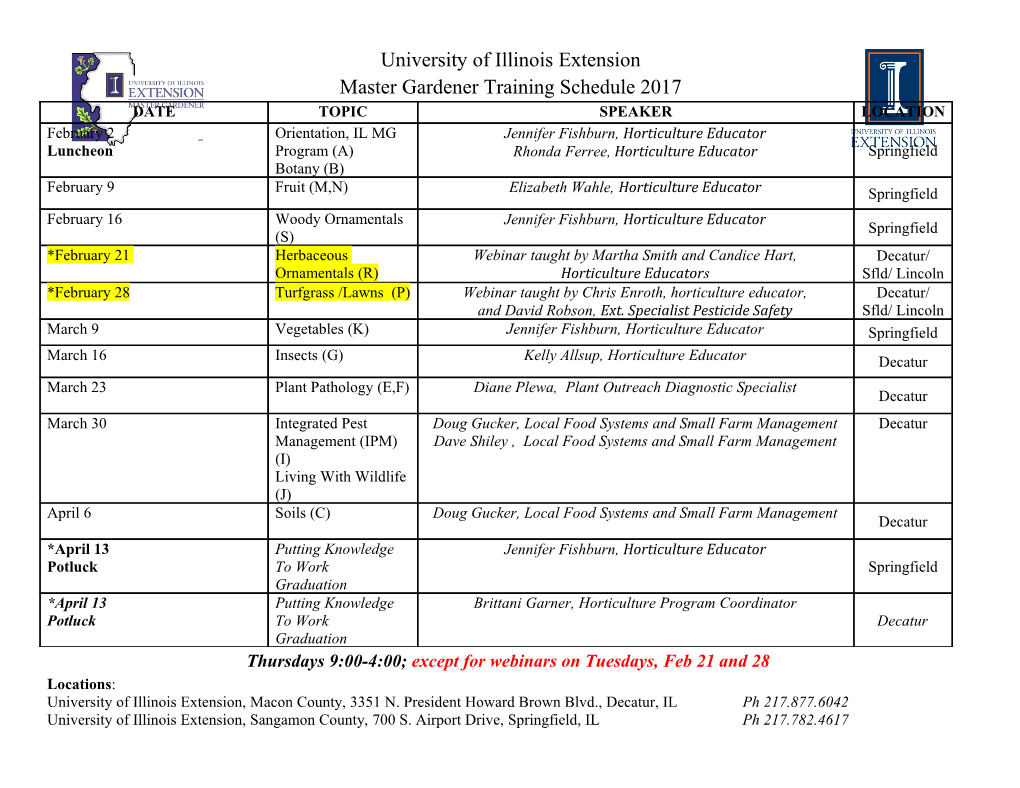
Today in Astronomy 111: the Kuiper Belt and the realm of the dwarf planets Pluto: second discovery of a ninth planet • Relation to Neptune and Uranus • Charon and the new moons The Kuiper Belt: undiscovery of the latest ninth planet Distribution and composition Artist-astronomer’s conception of KBOs of Eris, with the distant Sun at Notable KBOs: Eris, Quaoar lower right (Robert Hurt, and Haumea SSC/Caltech). Past the Kuiper Belt: Sedna 22 November 2011 Astronomy 111, Fall 2011 1 Pluto and Neptune After Neptune’s discovery, differences remained between the observed and calculated orbits of both Uranus and Neptune, that couldn’t easily be explained as perturbations by other known planets. Thus a search for another perturber – a massive trans-Neptunian planet – was launched. Percival Lowell – he of the Martian canals – was the most ardent seeker of that planet, using telescopes at a site near Flagstaff, AZ, now called Lowell Observatory . Years later Clyde Tombaugh was hired to carry on this work at Lowell Observatory. He followed up many dynamical-calculation predictions of the position of the perturbing planet with photographic searches for faint bodies that moved with respect to the fixed stars. 22 November 2011 Astronomy 111, Fall 2011 2 Pluto and Neptune (continued) There were many such calculations over the years, with results that varied widely. Eventually (on 18 February 1930), Tombaugh found X U Pluto, near the position N S predicted in one of these calculations. • This calculation, along with most of the others he followed up through the years, was seriously in error, as was immediately apparent by Pluto’s faintness. • Thus the discovery was accidental, and mostly a result of Tombaugh’s excellent observations. 22 November 2011 Astronomy 111, Fall 2011 3 Pluto and Neptune (continued) The Roman god of the underworld provided an appropriate name for such a distant, obscure solar system body (and also allowed commemoration of Percival Lowell). Tombaugh continued searching, eventually concluding that there were no other planets of Neptunian size in the ecliptic and closer to the Sun than 100 AU, and thus ruling out perturbers such as were theorized theretofore. Even more eventually, the methods with which to carry out the difficult orbital-perturbation calculations improved, and the precision with which we know the planetary orbits also improved. Result: essentially all of the anomalous differences between observations and theory went away. Nevertheless interest in whether there were massive trans-Neptunian planets remained, as astronomers sought a perturber to knock comets out of the Oort Cloud. 22 November 2011 Astronomy 111, Fall 2011 4 25 Mass 1.25× 10 gm (0.0021M⊕ ) Pluto’s vital 8 Equatorial radius 1.195× 10 cm (0.187R⊕ ) statistics Average density 1.75 gm cm-3 Bond albedo 0.5 5.90638× 1014 cm Orbital semimajor axis (39.482 AU) Orbital eccentricity 0.2488 Obliquity 122.53° Sidereal 247.68 years revolution period Sidereal -6.38725 days rotation period Pluto and Charon, Moons 4 so far from HST (R. Albrecht, Surface temperature ~40 K ESA/NASA) 22 November 2011 Astronomy 111, Fall 2011 5 Pluto and Neptune (continued) Pluto’s orbit has larger semimajor axis, eccentricity and inclination than the orbits of any of the eight planets. Its orbit takes it closer to the Sun than Neptune. In fact it was the “eighth planet” in this regard fairly recently (1979-1999). Although its orbit crosses Neptune’s in projection, the orbits don’t intersect, and it never gets very close to Neptune. In fact it gets closer to Uranus than it ever gets to Neptune. • This is due to orbital dynamics: Neptune and Pluto are locked in a 3:2 mean-motion orbital resonance, as you hopefully noticed in Homework #4, problem 5b. 22 November 2011 Astronomy 111, Fall 2011 6 Pluto and Neptune (continued) The orbits of Pluto and the giant planets, in an inertial frame of reference (left), and in a frame rotating with the revolution of Neptune (right), for a time span very long compared to Pluto’s orbital period. (Calculation by Renu Malhotra and Jeff Williams.) Note that Pluto never gets nearly as close to Neptune as its orbit gets to Neptune’s orbit, a result of their 3:2 resonance. 22 November 2011 Astronomy 111, Fall 2011 7 Pluto and Charon In 1978 Pluto was discovered, by Christy and Harrington, to have a moon, dubbed Charon after the Styx boatman. Measurement of the revolution of the two, and Kepler’s laws, provided the first good estimate of the mass of Pluto (and Charon). Previous estimates were based upon guesses for its albedo. From 1985 to 1990, Pluto and Charon eclipsed each other frequently, from which their sizes (and thus densities) could be deduced. Results: Pluto turned out to be smaller, and much higher in albedo, than previously thought. Pluto is considerably smaller than the Earth’s Moon, not to mention Titan, Triton and the Galilean satellites of Jupiter. 22 November 2011 Astronomy 111, Fall 2011 8 Pluto and Charon (continued) Charon turns out to be about half the diameter, and about one-eighth the mass, of Pluto itself, and the two have a relatively small separation (19,600 km). • Their center of mass lies outside the bounds of both bodies, the only major “double” in the Solar system known to have this property. • They are completely tidally locked, in the sense that both Pluto and Charon have rotation periods equal to their orbital period, 6.4 days; they always show each other the same face. • The two have similar densities. Both have large and albedoes that vary over their surfaces, indicating some differentiation and an icy composition. • Obliquity >90°: the rotation is retrograde. 22 November 2011 Astronomy 111, Fall 2011 9 Pluto and Charon (continued) Above: views of Pluto centered on longitudes 270°, 180° and 90° (left-right). Left: full globe. By Marc Buie, with the Hubble Space Telescope (SwRI/ESA/NASA) 22 November 2011 Astronomy 111, Fall 2011 10 Pluto and Charon (continued) Pluto has an atmosphere, revealed in the usual outer- planet manner by stellar occultation and spectroscopy. • Composed mostly of nitrogen and methane, like the atmosphere of Triton. • Pretty thin (pressure 10 − 6 standard Earth atmospheres), also like the atmosphere of Triton. In its gross properties, Pluto seems a lot like Triton, which we have of course seen at close range. Our first chance to see Pluto at close range will come with the New Horizons (“Pluto-Kuiper Express”) mission, which was launched early January 2006 and will reach Pluto in 2015. 22 November 2011 Astronomy 111, Fall 2011 11 Pluto and Charon (continued) Three additional moons, very small ones, have been detected in the Pluto-Charon system: Nix and Hydra both in 2005, and S/2011 P4 this past July. All revolve around the system’s center of mass in the same direction Pluto and Charon do. Showalter et al. 2011, SETI Institute/ STScI/NASA 22 November 2011 Astronomy 111, Fall 2011 12 The Kuiper Belt Speculation on the origin of short-period comets has always focussed on the region just outside Neptune’s orbit. In 1943 Edgeworth suggested – briefly, vaguely, and mostly incorrectly – that comet-like bodies might be concentrated there. Later this was also suggested, similarly briefly and vaguely, by Kuiper (1951). The discovery of a belt of such objects, beginning in 1992 with observations by Dave Jewitt and Jane Luu, began rather than completed the tale. Now called the Kuiper Belt, there are currently 1585 members (called Kuiper Belt Objects, or KBOs) known, mostly discovered recently as large CCD detector arrays and large telescopes have enabled searches far from the ecliptic plane. If life were fair, its name would be the Jewitt-Luu Belt. 22 November 2011 Astronomy 111, Fall 2011 13 Characteristics of KBOs Most KBOs are found with a = 37-47 AU. There are probably about 100,000 of them of substantial (> 100 km) size. They have a wide range of colors, going all the way from colors similar to very icy bodies (e.g. Chiron) to those of very red, carbonaceous bodies (e.g. Pholus). • Inside they’re still expected to be icy, but they can be “carbonized” on the outside: covered with lots of dark, probably organic, material. • This material can be produced from carbon originally in the ice (as CH4, CH3OH, H2CO,…) due to prolonged exposure to cosmic rays, the solar wind, and solar ultraviolet radiation. 22 November 2011 Astronomy 111, Fall 2011 14 Colors of KBOs From Jewitt and Luu 1996. Chiron’s color is like that of Saturn’s iciest moons. Pholus is the same color as Mars and the redder asteroids. 22 November 2011 Astronomy 111, Fall 2011 15 Sorting the KBOs Mean-motion 5:4 7:4 Curves of constant orbital resonances 4:3 5:3 perihelion distance, with Neptune: 1:1 3:2 2:1 5:2 rap =(1: −ε ) 30 AU 35 AU 40 AU 45 AU Figure by Eugene Chiang (see Chiang et al. 2007) 22 November 2011 Astronomy 111, Fall 2011 16 Sorting the KBOs (continued) Classical KBOs (, 47%) are rather red as KBOs go, and live in low- eccentricity, low- inclination orbits. The edges of their distribution are rather sharp at a = 37 AU and 47 AU, the latter lining up with the 2:1 Neptune mean-motion resonance. None have planet- crossing orbits. 22 November 2011 Astronomy 111, Fall 2011 17 Sorting the KBOs (continued) Resonant KBOs (, 23%) stably occupy the mean-motion orbital resonances with Neptune. The most numerous of these, at 60% of the total, are the plutinos (locked in the 3:2 resonance), which include Pluto and Orcus.
Details
-
File Typepdf
-
Upload Time-
-
Content LanguagesEnglish
-
Upload UserAnonymous/Not logged-in
-
File Pages34 Page
-
File Size-