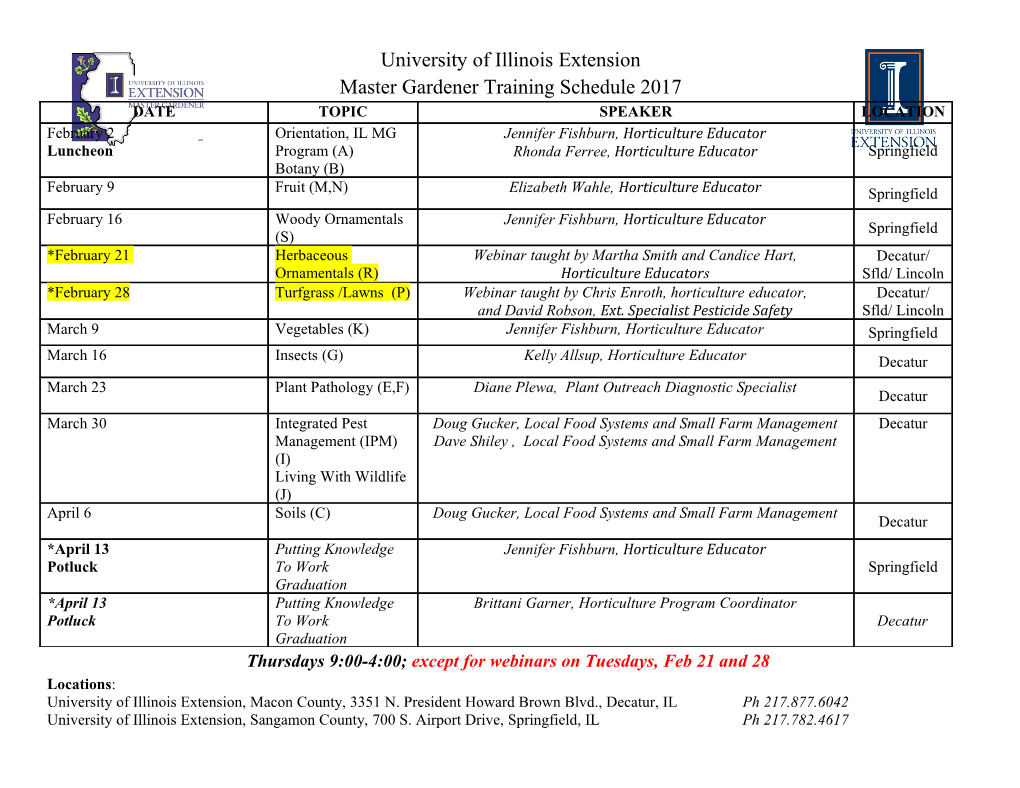
AAAS Research Volume 2020, Article ID 7304767, 10 pages https://doi.org/10.34133/2020/7304767 Research Article Structure-Enhanced Mechanically Robust Graphite Foam with Ultrahigh MnO2 Loading for Supercapacitors Qinghe Cao,1 Junjie Du,1 Xiaowan Tang,1 Xi Xu ,2 Longsheng Huang,3 Dongming Cai,3 Xu Long ,4 Xuewen Wang,1 Jun Ding,2 Cao Guan ,1 and Wei Huang 1 1Frontiers Science Center for Flexible Electronics, Institute of Flexible Electronics, Northwestern Polytechnical University, Xi’an 710072, China 2Department of Materials Science and Engineering, National University of Singapore, 9 Engineering Drive 1, Singapore, Singapore 117576 3College of Chemical Engineering, Hubei University, Wuhan 430062, China 4School of Mechanics, Civil Engineering and Architecture, Northwestern Polytechnical University, Xi’an 710072, China Correspondence should be addressed to Xi Xu; [email protected], Cao Guan; [email protected], and Wei Huang; [email protected] Received 25 August 2020; Accepted 19 October 2020; Published 10 November 2020 Copyright © 2020 Qinghe Cao et al. Exclusive Licensee Science and Technology Review Publishing House. Distributed under a Creative Commons Attribution License (CC BY 4.0). With the fast bloom of flexible electronics and green vehicles, it is vitally important to rationally design and facilely construct customized functional materials with excellent mechanical properties as well as high electrochemical performance. Herein, by utilizing two modern industrial techniques, digital light processing (DLP) and chemical vapor deposition (CVD), a unique 3D hollow graphite foam (HGF) is demonstrated, which shows a periodic porous structure and robust mechanical properties. Finite element analysis (FEA) results confirm that the properly designed gyroidal porous structure provides a uniform stress area and mitigates potential structural failure caused by stress concentrations. A typical HGF can show a high Young’s modulus of -3 3.18 MPa at a low density of 48.2 mg cm . The porous HGF is further covered by active MnO2 material with a high mass -2 -3 fi -1 loading of 28.2 mg cm (141 mg cm ), and the MnO2/HGF electrode still achieves a satisfactory speci c capacitance of 260 F g , corresponding to a high areal capacitance of 7.35 F cm-2 and a high volumetric capacitance of 36.75 F cm-3. Furthermore, the assembled quasi-solid-state asymmetric supercapacitor also shows remarkable mechanical properties as well as electrochemical performance. 1. Introduction ing technology, it has been widely utilized for the construc- tion of functional materials with unique predesigned The growing requirement for energy in electronics as well as structures for efficient energy storage devices [12–19]. For vehicles has prompted extensive researches on the develop- example, 3D graphene/graphite-based materials have been ment of high-performance energy storage devices with widely studied for high-performance energy storage devices higher energy densities and power densities [1–4]. Increasing due to their low density, high conductivity, and excellent − the mass loading (larger than 10 mg cm 2) for commercially electrochemical stability [20–24]. However, most of the pre- available electrodes often leads to the decline of utilization vious studies on 3D printed electrode materials were focused efficiency of the active materials due to sluggish ion/electron on extrusion-based techniques, where the resolution is low transport in bulk reactions [5–11]. Design of a 3D structured (usually larger than 200 μm) and only certain simple 3D electrode containing interconnected porous network can structures (like lattices and interdigitated structure) can be ensure efficient charge transport throughout the entire elec- achieved. In addition, the mechanical properties of such 3D trode, which is necessary for the utilization of all active mate- carbon materials, which are essential for packaging, trans- rials and the realization of high rate capability with high portation, and utilization, are also important for the realiza- capacity/capacitance. With the fast development of 3D print- tion of high-performance energy storage devices, but they 2 Research 3D printer HGF MnO2/HGF SiO2 Polymer removed Graphite growth MnO2 growth & SiO2 sintered & etching Figure 1: Schematic illustration of the fabrication process of the MnO2/HGF electrode. were merely discussed in previous reports [11, 15, 18, 24–27]. details are in the Supplementary Materials (available here)). fi With the above concern, it will be very promising to develop A gyroid SiO2 template was rstly designed by computer novel 3D printed electrodes with higher resolution and software and prepared by the high-resolution DLP technol- unique structure design, which will bring about promising ogy with photopolymerization of UV-curable resin mixed mechanical properties and electrochemical performance. with SiO2 microspheres. After 3D printing, a debinding and In this work, an ultralight and ultrastiff HGF with a sintering process was further conducted, in which the photo- gyroid structure is prepared though integrating DLP technol- polymer was completely removed and SiO2 was molded. ogy with the CVD method. Different from extrusion-based Then, a graphite layer was deposited on the surface of the 3D printing, DLP technology is based on a fast photopoly- SiO2 template by the CVD method (the experimental setup merization process where few structural supports are and reaction route diagram are shown in Figure S1), where required; thus, various complex 3D architectures with high the densities and mechanical properties of the graphite can resolution can be achieved [28–31]. The selected gyroid be simply controlled by the deposition time. After etching ff structure has been reported with high sti ness at low density, away the SiO2 by aqueous HF solution, MnO2 nanosheet but it is hard to construct it with extrusion-based methods arrays were further grown on the surface of HGF, resulting fi [24, 26]. FEA results con rm that the gyroid HGF can pro- in a hybrid electrode of MnO2/HGF. vide a uniform stress distribution, thereby mitigating early structural failure caused by stress concentrations. The final 2.2. The Mechanical Properties of HGF. FEA is performed to HGF can show a high Young’s modulus of 3.18 MPa at the evaluate the influences of the structure for HGF on their low density of 48.2 mg cm-3 in compressive performance mechanical properties (Figure 2(a)). For comparison, three tests. In addition to the mechanical robustness, the HGF elec- shell structures including lattice, primitive, and gyroid were trode with well-designed hierarchical porosity also can modeled and analyzed, and their volume (2×2×1mm3) fi deliver a high mass loading of MnO2 with superior electro- and thickness (0.1 mm) are xed consistently. (Red color chemical performance. MnO2/HGF with a high mass loading highlights the stress concentration area. According to the of 28.2 mg cm-2 (141 mg cm-3) achieves a high areal capaci- barrel principle, the fracture of these stress concentration tance of 7.35 F cm-2 and a high volumetric capacitance of points will lead to the destruction of the overall structure.) 36.75 F cm-3 with a specific capacitance of 260 F g-1. Further As indicated from the color distribution, the gyroid structure assembled HFG-based quasi-solid-state asymmetric superca- can provide a uniform stress area with no significant red part. pacitor also exhibits a remarkable energy density of This can be attributed to the continuous periodic structure 0.96 mWh cm-2 under a power density of 50 mW cm-2 as well that can relive stress well, thus effectively avoiding the possi- as excellent mechanical stability. The 3D HGF with hierar- ble collapse caused by the localized stress concentration. As a chical porous structure and robust mechanical properties, comparison, multiple stress concentration points appeared at the customizable facile fabrication process using 3D printing the connection parts for lattice structure and the neck regions and CVD, together with the promising mechanical and elec- for primitive structure, indicating poor mechanical stability. trochemical properties would pave a good way for the devel- In order to further explore the mechanical properties, a − opment of high-performance energy storage devices. gyroidal HGF with an ultralow density of 48.2 mg cm 3 was prepared. As demonstrated in Figure 2(b), the HGF with a 2. Results volume of 2:7 ∗ 1 ∗ 0:2cm3 can steadily stand on a dandelion flower without causing any deformation. More important, 2.1. Fabrication of MnO2/HGF Electrode. The schematic fab- the ultralight HGF can withstand 15000 times its own weight rication process of the MnO2/HGF electrode with the photo- without obvious degradation (Figure 2(c)). To further con- graphs of the samples at each step is shown in Figure 1 (more firm, a compressive test is carried out and the stress-strain Research 3 Compressive stress �/� �/�Max Max �/�Max 1.00 1.00 1.00 z 0.93 0.51 0.45 y 0.89 0.20 0.02 x Lattice Primitive Gyroid (a) 200 g 13.2 mg (b) (c) 1.00 1.6 8 0.75 1.2 6 0.50 0.8 4 0.25 0.4 2 Modulus (MPa) Modulus 0.00 0.0 0 Compressive strengthCompressive (MPa) Compressive strengthCompressive (MPa) 0102030 48.2 56.5 66.3 95.675.3 Compressive strain (%) Density (mg cm−3) 48.2 mg cm−3 56.5 mg cm−3 66.3 mg cm−3 75.3 mg cm−3 95.6 mg cm−3 (d) (e) Figure 2: Mechanical properties of HGF. (a) FEA models of lattice, primitive, and gyroid structures and their stress distributions under the z σ same compressive strain along the -direction (the values of Max for lattice, primitive, and gyroid structure are 600 MPa, 770 MPa and 600 MPa, respectively). (b) Ultralight and (c) Ultrastiff properties illustrated by the gyroidal HGF. (d) Compressive stress-strain curves of HGF with different densities.
Details
-
File Typepdf
-
Upload Time-
-
Content LanguagesEnglish
-
Upload UserAnonymous/Not logged-in
-
File Pages10 Page
-
File Size-