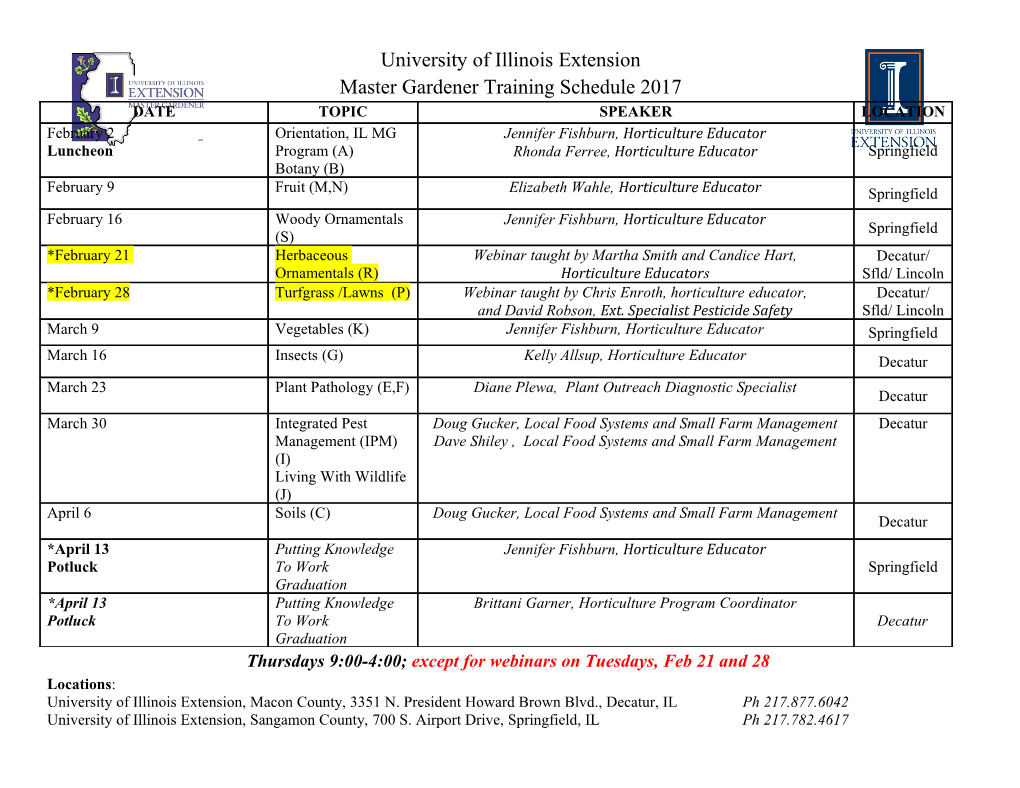
1JANUARY 2000 HAERTEL AND JOHNSON 93 The Linear Dynamics of Squall Line Mesohighs and Wake Lows PATRICK T. H AERTEL AND RICHARD H. JOHNSON Department of Atmospheric Science, Colorado State University, Fort Collins, Colorado (Manuscript received 22 September 1998, in ®nal form 16 April 1999) ABSTRACT Two surface pressure features that commonly accompany squall lines, the mesohigh and the wake low, may be explained at least in part as a linear response to the low-level cooling associated with stratiform precipitation. This response is numerically simulated for two- and three-dimensional, moving and stationary low-level coolings characteristic of squall line stratiform regions. When the cooling is de®ned to move and to have three-dimensional structure both a mesohigh and a mesolow develop, and their structures and evolutions resemble those of squall line mesohighs and wake lows. When an upper boundary is introduced directly above the cooling the response approaches a steady state in which a mesohigh±mesolow couplet is centered on the cooling. The simulations and the steady-state analysis presented here help to explain observed characteristics of squall line mesohighs and wake lows, including their life cycles and positioning relative to precipitation structures. 1. Introduction imum intensity. Finally, the wake low ®lls during the remnant stage (not shown). Pedgley (1962) observed a a. Background similar life cycle within squall systems over Great Brit- Much of the springtime rain over the central United ain, and Johnson and Hamilton (1988) also observed a States comes from systems that include a propagating squall system whose surface pressure features exhibited line of vigorous convection, or squall line, and a trailing this life cycle. region of stratiform precipitation (Houze et al. 1990). The structure of surface pressure within a squall sys- Two intense surface pressure features referred to as the tem is closely related to the pattern of precipitation. mesohigh and the wake low typically accompany such Loehrer and Johnson (1995) examined this relationship a system (Fujita 1955; Pedgley 1962; Williams 1963; for 16 systems that passed over the central United States Johnson and Hamilton 1988; Stumpf et al. 1991; Loeh- during May and June 1985. They constructed schemata rer and Johnson 1995). Figure 1 depicts radar re¯ectiv- for two radar-re¯ectivity patterns identi®ed by Houze ity, surface pressure, and surface winds within a mature et al. (1990; Fig. 3). For the symmetric system the en- squall system with the leading-line/trailing-stratiform hanced stratiform precipitation lies directly behind the organization. The mesohigh is centered just behind the convective line. For the asymmetric system the strati- convective line, and the wake low is centered on a strong form region is displaced to the left of the direction of gradient in re¯ectivity on the trailing edge of the strat- propagation. For both systems the mesohigh±wake low iform region. couplet is approximately centered on the stratiform re- Mesohighs and wake lows evolve during a squall sys- gion. tem's lifetime. Fujita (1955, 1963) identi®ed ®ve stages Both mesohighs and wake lows are primarily hydro- in a life cycle, four of which are depicted in Fig. 2. A static in nature. Above the mesohigh the lower tropo- weak mesohigh forms during the initiation stage, and sphere is cool and dense (Fujita 1959; Johnson and it grows in scale and intensity during the development Hamilton 1988; Smull and Jorgensen 1990) and its ex- stage. Showers reach their maximum intensity during cess weight per area accounts for most of the pressure the mature stage and the wake low forms behind them. surplus (Fujita 1959). This coolness has been attributed During the dissipation stage the precipitation and the to the evaporation, melting, and sublimation of hydro- mesohigh diminish, but the wake low reaches its max- meteors (Fujita 1959; Zhang and Gao 1989; Gallus 1996). While such phase changes of atmospheric water cool air locally, they also cause parcels to become neg- atively buoyant, to descend, and to transport the cool- Corresponding author address: Dr. Patrick Haertel, Department of Atmospheric Science, Colorado State University, Fort Collins, CO ness downward (Rotunno et al. 1988). Gallus and John- 80523-1371. son (1991) measured the lower-tropopheric cooling (dia- E-mail: [email protected] batic forcing) resulting from all of these processes both q 2000 American Meteorological Society Unauthenticated | Downloaded 09/28/21 03:29 AM UTC 94 JOURNAL OF THE ATMOSPHERIC SCIENCES VOLUME 57 FIG. 1. Schema of a mature squall system (adapted from Johnson and Hamilton 1988). Surface pressure is contoured, vectors represent surface wind, and signi®cant regions of precipitation are stippled. in the convective line and the stratiform region of a a consensus that this lower-tropospheric warmth results squall system, and they found that while there was cool- from subsidence, but there are different ideas regarding ing in both places, the stratiform cooling was deeper the cause of the subsidence. Miller and Betts (1977) and more intense. Other factors that can contribute to suggested that the subsidence is dynamically driven by the mesohigh include the weight of hydrometeors (Sand- the spreading of cold air near the surface. Johnson and ers and Emanuel 1977) and nonhydrostatic pressure Hamilton (1988) connected the subsidence to rear in¯ow (Schaffer 1947). into the system. Zhang and Gao (1989) called the wake The de®cit in pressure within the wake low approx- low an ``end product of a chain of complicated dynamic imately equals the de®cit in weight per area of a warm, reactions'' associated with latent cooling. Schmidt and not-so-dense lower troposphere (Williams 1963; John- Cotton (1990) attributed the subsidence and warming son and Hamilton 1988; Stumpf et al. 1991). There is to gravity waves. Gallus (1996) showed that micro- FIG. 2. Contours of surface pressure for four stages of a squall system (from Fujita 1963). The W denotes warm sector, i.e., the system formed on the warm side of a front. Unauthenticated | Downloaded 09/28/21 03:29 AM UTC 1JANUARY 2000 HAERTEL AND JOHNSON 95 FIG. 3. Schemata of symmetric and asymmetric squall systems. Levels of shading denote increasing radar re¯ectivity, surface pressure is contoured with a 1-hPa interval, and vectors represent surface winds (from Loehrer and Johnson 1995). physical processes within the stratiform region alone and in turn apply this understanding to squall line me- could generate strong subsidence, but only when the sohighs and wake lows. precipitation rates were prescribed to decrease with time The numerical model is described in section 2, section did the subsidence generate signi®cant lower-tropo- 3 contains the simulations, the steady-state analysis is spheric warming. presented in section 4, and section 5 is a summary and discussion. b. Motivation 2. The numerical model While previous studies have provided much infor- mation about squall line mesohighs and wake lows, their a. The equations description of these surface pressure features is still lacking. They neither clarify the cause of the subsidence The numerical model is based on the hydrostatic responsible for the wake low, nor do they explain the Boussinesq equations linearized about a basic state of observed life cycles of mesohighs and wake lows (Fig. rest with a rigid upper boundary and no Coriolis force: 2) or their positioning relative to precipitation structures ]D 1 (Fig. 3). In this paper we provide plausible explanations 1¹2p 5 0 (1) for each of these phenomena using linear dynamics. ]t r0 Using a simple numerical model we show that a portion 1 ]p of a squall system's thermal forcing, the low-level cool- 5 b (2) ing associated with stratiform precipitation, can generate r0 ]z a linear response that includes a mesohigh±mesolow ]b couplet similar to that observed in squall systems. We 1 wN2 5 Q (3) also present a steady-state analysis that predicts that ]t such a couplet will develop in response to a moving, ]w D 150, (4) three-dimensional low-level cooling. Because of the ide- ]z al nature of the numerical simulations and the analysis presented here, one can fully understand their dynamics where ¹ 2 is the horizontal Laplacian, D is horizontal Unauthenticated | Downloaded 09/28/21 03:29 AM UTC 96 JOURNAL OF THE ATMOSPHERIC SCIENCES VOLUME 57 23 divergence, r 0 is the basic-state density (1.0 kg m ), of how the atmosphere responds to each and all of these p is perturbation pressure, b is buoyancy, w is vertical components of thermal forcing is an interesting one, for velocity, N is the buoyancy frequency (0.01 s21), and this paper we have decided to focus on the response to Q is the thermal forcing. At the lower and upper bound- the lower-tropospheric cooling associated with strati- aries, w vanishes. form precipitation. Haertel and Johnson (1998) showed The linearization of (1)±(4) removed terms related to that the stratiform cooling has a much greater impact the vertical and horizontal advection of pertubation wind on surface pressure than either the convective or strat- and buoyancy. For a consistency check we calculated iform heating, and the results presented here establish the magnitude of these terms within the ®rst simulation that the response to the stratiform cooling can account presented here and found them to be about half as in- for much of the surface pressure structure in the squall tense as the related linear terms. Based on this calcu- systems. We do not, however, assess the contribution of lation we conclude that while the linear approximation the convective cooling, which might also signi®cantly is consistent, we would expect nonlinear simulations to alter surface pressure. Unlike the stratiform cooling it exhibit noticeable differences.
Details
-
File Typepdf
-
Upload Time-
-
Content LanguagesEnglish
-
Upload UserAnonymous/Not logged-in
-
File Pages15 Page
-
File Size-