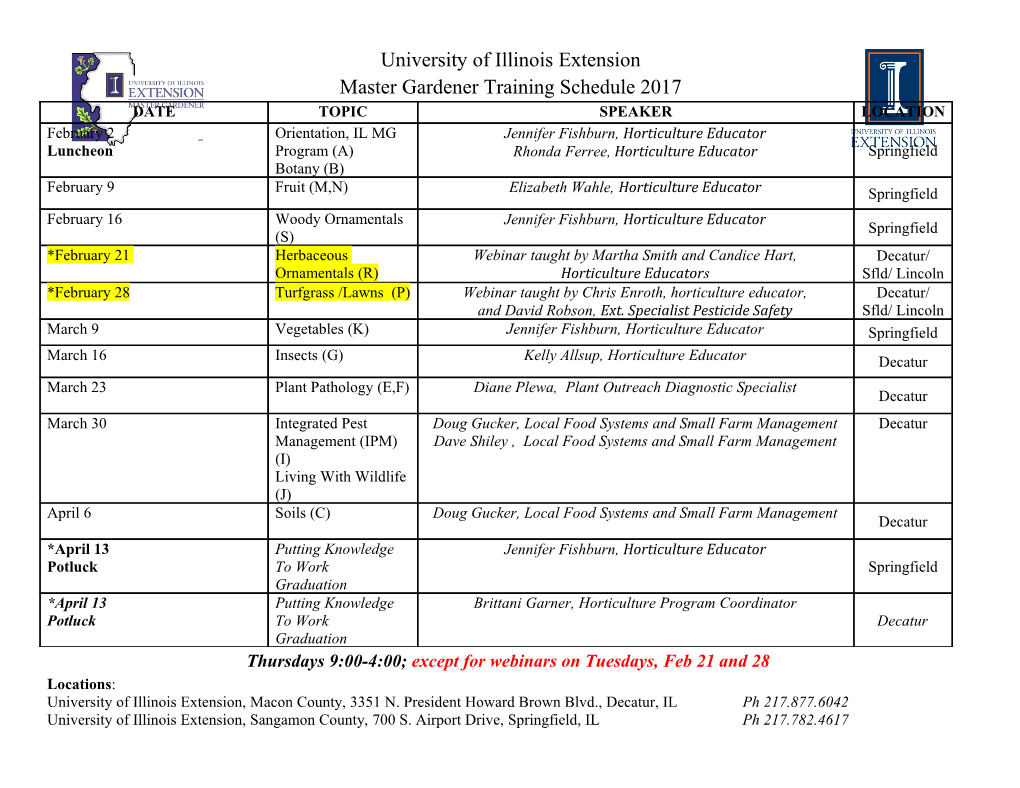
Practical Limitations on Astrophysical Observations of Methanol to Investigate Variations in the Proton-to-Electron Mass Ratio Simon Ellingsen1, Maxim Voronkov2, and Shari Breen2 1School of Mathematics and Physics, Private Bag 37, University of Tasmania, TAS 7001, Australia∗ and 2CSIRO Astronomy and Space Science, PO Box 76, Epping NSW 1710, Australia (Dated: November 5, 2018) The possibility of using astrophysical observations of rotational transitions in the methanol molecule to measure, or constrain temporal and spatial variations in the proton-to-electron mass ratio (µ) has recently been investigated by several groups. Here we outline some of the practical considerations of making such observations, including both the instrumental and astrophysical lim- itations which exist at present. This leads us to conclude that such observations are unlikely to be able to improve evidence either for, or against the presence of variations in the proton-to-electron mass ratio by more than an order of magnitude beyond current limits. PACS numbers: 06.20.Jr, 33.15.-e, 98.80.-k, 98.38.Er, 98.58.Ec The possibility of using astrophysical observations of ter than 100 ms−1. When the 6.7 and 12.2 GHz methanol rotational transitions in the methanol molecule to mea- transitions exhibit peaks at the same velocity they have sure, or constrain temporal and spatial variations in the been demonstrated to arise from the same locations at proton-to-electron mass ratio (µ) has recently been sug- the milliarcsecond level (corresponding to linear scales of gested by several groups [1, 2]. The first attempts to a few AU at distances of a few kpc) [6, 7]. The large num- use astronomical observations of methanol masers to con- ber of 6.7 and 12.2 GHz methanol masers observed within strain µ within the Milky Way show that |∆µ/µ| < the Milky Way makes them potentially useful for prob- 28 × 10−9 [2]. ing spatial variations in µ. Perhaps more significantly, The hindered internal rotation exhibited by methanol emission from these two transitions in external galaxies produces the degeneracy which makes the different ro- may allow temporal/cosmological evolution to be inves- tational transitions particularly sensitive to the proton- tigated. However, there are two practical issues which to-electron mass ratio [1]. It also leads to the rich rota- need to be considered: 1. The degree to which the emis- tional and vibrational spectrum, which due to quantum sion from the two transitions is coincident/cospatial and mechanical selection rules produces a large number of 2. The likelihood of being able to detect emission from centimetre and millimetre maser transitions in interstel- these transitions at cosmological distances. We address lar space. The strongest and most common of these is each of these issues in turn below. + the 51 → 60A transition which has a rest frequency The requirement for using astrophysical observations of approximately 6.7 GHz. It has been detected to- of different methanol transitions to measure spatial or wards more than 900 sites of high-mass star formation temporal changes in µ is that you can measure the veloc- in the Milky Way [see for example 3, 4]. The second ity (frequency) offset for the two transitions, compared strongest astrophysical transition of methanol is from the to the laboratory values, and that the observed shift 20 → 3−1E transition which has a rest frequency of ap- is due to a change in µ. Levshakov et al. [2] identi- proximately 12.2 GHz, and is observed towards 43% of fied that intrinsically strong and narrow spectral lines those sources showing 6.7 GHz emission [5]. In most (e.g. interstellar masers) provide the best opportunity to arXiv:1111.4708v1 [astro-ph.CO] 21 Nov 2011 methanol maser regions multiple spectral features are ob- achieve this, but suggested uncertainties in the rest fre- served in the spectra of 6.7 or 12.2 GHz methanol masers quencies of the transitions as the major source of error. at different Doppler shifted velocities [3, 4]. The spectral We first review the extensive observational information features typically have near-Gaussian profiles (although on methanol masers which has been gathered, primarily spectral blending between spatially unresolved compo- over the last 20 years, to determine if there are other nents affects most observations), and FWHM (full-width possible causes for differences between the observed peak half maximum) of a few tenths of kilometers per second. velocity of methanol maser transitions from the same re- gion, and their likely magnitude compared to differences The Kµ coefficient measures the sensitivity of a tran- sition to the proton-to-electron mass ratio and for the caused by variations in µ. 6.7 and 12.2 GHz methanol transitions these have been The 6.7 and 12.2 GHz transitions of methanol are calculated to be -42 and -33 respectively [1]. To improve members of the A and E rotational species respectively. constraints on |∆µ/µ|, beyond those already achieved [2], Although chemically identical, they each have indepen- requires measurement of the relative Doppler shifted ve- dent rotational spectra and are expected to have com- locities of the different transitions to an accuracy of bet- parable abundances in interstellar molecular clouds, the 2 most extreme difference in abundance is expected to be 6.7 GHz. approximately 40% [8]. At high angular resolution the The precise geometry of the masers is not known, how- 6.7 and 12.2 GHz methanol masers are observed to have ever, a picture self-consistent with the available observa- a complex spatial morphology, with emission present on tional evidence is that the maser spots are regions within multiple scale sizes [9, 10]. The maser emission is typi- the turbulent molecular gas, where by chance there is an cally observed as a series of “spots” with bright cores of unusually high fraction of the material with velocity co- a few-10 AU, surrounded by weaker emission on scales herence along our particular line of sight [see fig. 7c of of 10s-100s of AU [9], but sometimes extending to 1000s 9]. Under this model, an observer along a different line- of AU [10]. The maser spots usually arise in clusters of-sight is likely to also detect maser emission from the with linear scales of 6000 AU [11]. There are relatively same region, but from different parts of the cloud, and few cases where the spatial structure of individual maser the linear size of the maser cluster gives a reasonable es- spots has been investigated in different coincident tran- timate of both the size of the maser-conducive region and sitions towards the same source, however, where they the maximum path length. Also, the relative intensity of have it has been found that the structure as measured the 6.7 and 12.2 (or any other pair of transitions) from a by plots of the visibility versus baseline length differ particular maser spot will depend critically upon the spe- [9]. This indicates that the spatial scales of the emission cific physical conditions in the velocity coherent fractions from the two “coincident” transitions differs. Individual of the path. These are likely to vary between the various methanol maser spots are also observed to have internal disjoint regions of the path through the molecular gas velocity gradients, sometimes in different directions from which produces the maser. Breen et al. [14] show that the larger scale gradients seen between individual spots the variation in the 6.7 versus 12.2 GHz maser luminosity within a particular region [12, 13]. Very high spatial is much less between maser spots/spectral features within − (few AU) and spectral (20 ms 1) resolution observations an individual source than it is between different star for- of the 12.2 GHz methanol masers in W3(OH) measure mation regions. It is also observed that while the variabil- − − velocity gradients of 20-300 ms 1AU 1, and show devi- ity of 6.7 GHz methanol masers on timescales of decades ations from a Gaussian profile are typically at levels less frequently causes the velocity of the peak spectral fea- than 0.2% at these spatial resolutions [12]. ture to change, this comes about through the changes In the presence of turbulence within the masing gas, if in the relative intensities of different features which were the physical conditions were homogeneous, and multiple present over the whole period [3, 4, 16]. It is also clear maser transitions were saturated and completely cospa- that the the peak intensity of the stronger masers usually tial, then the spectra from the different transitions would changes by less than 50%, which is consistent with the be identical except for a single scaling factor. What is intensity of the maser features being governed primarily observed though, is that the spectra of 6.7 and 12.2 GHz by the physical conditions over a region much larger than masers towards the same regions are usually significantly the scale size of individual maser spots. different [see for example 14]. While in many cases emis- Methanol is a diamagnetic molecule, so in the presence sion is observed from both transitions for many spectral of a magnetic field it experiences weak Zeeman splitting. features, and the peak emission is at the same veloc- Vlemmings et al.[17] measured a mean velocity difference − ity 80% of the time, the ratio of the intensity for differ- of 0.6 ms 1 between the two circular polarizations in a ent spectral features of the two transitions varies greatly sample of 44 6.7 GHz methanol masers. So the effects of (usually by more than an order of magnitude) within a the magnetic fields in the star formation region on the single source [14].
Details
-
File Typepdf
-
Upload Time-
-
Content LanguagesEnglish
-
Upload UserAnonymous/Not logged-in
-
File Pages5 Page
-
File Size-