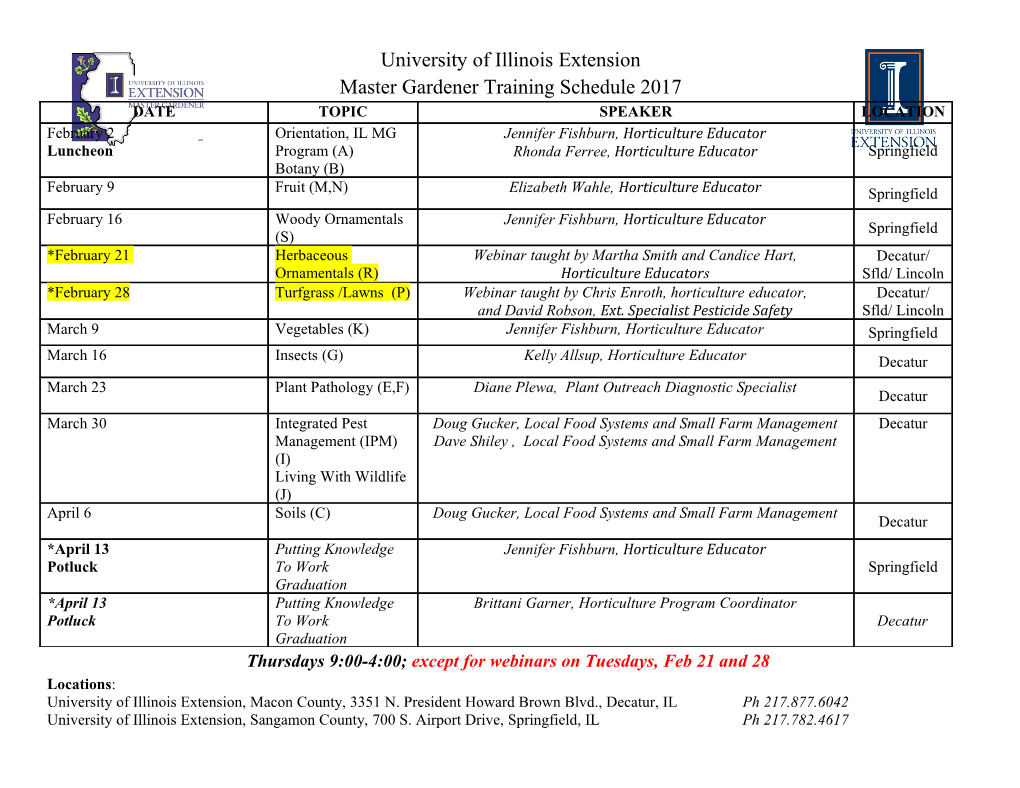
Intensity Correlations of Light Scattered From Cold Atom Clouds A thesis submitted to the Miami University Honors Program in partial fulfillment of the requirements for University Honors by Matthew Beeler May, 2003 Oxford, Ohio ii ABSTRACT INTENSITY CORRELATIONS OF LIGHT SCATTERED FROM COLD ATOM CLOUDS We have constructed an atom trap using laser light and magnetic fields. We confine approximately 10 million atoms to a 1 cubic mm space at a temperature on the order of 100 microKelvin. At a great enough trap density, radiation trapping begins to occur inside the trap. Radiation trapping is the reabsorption by atoms in the trap of spontaneously emitted photons from other atoms in the trap. Radiation trapping lowers the density and raises the temperature of cold atom traps. Because it is a result of spontaneous emission, radiation trapping will decohere the light scattered from the trap. Measurement of the intensity correlation function will show the effect of radiation trapping. The intensity correlation function is simply the probability of detecting two photons separated by a certain time delay. A theoretical exploration of the effect of radiation trapping on the intensity correlation function is made. We show that the effects of radiation trapping are significant at trap densities two orders of magnitude less than previously thought. Experimental progress on verifying our predictions is reported. iii iv Intensity Correlations of Light Scattered From Cold Atom Clouds By Matthew Beeler Approved By: Dr. Samir Bali, Advisor Dr. Perry Rice, Reader Dr. Michael Pechan, Reader Accepted By: Dr. Carolyn Haynes, Director, University Honors Program v vi Acknowledgements I would like to acknowledge my advisor Dr. Samir Bali, without whom none of this would have been possible. Also, I would like to thank the people that I have worked closely with over the last three years: Soo Kim, Ron Stites, Laura Feeney, and Kristy Kallback-Rose. We have also received help from Dr. Perry Rice and Dr. S. Douglas Marcum, and I would like to thank Dr. Michael Pechan and Dr. Perry Rice for being readers of this thesis. Financial support for this project was provided by the College of Arts and Science, the Office for the Advancement of Scholarship and Teaching (OAST), and Miami University. Also, support from the Cottrell Foundation (Research Corporation) and the Petroleum Research Fund of the American Chemical Society is appreciated. A paper entitled “Sensitive Detection of Radiation Trapping Cold Atom Clouds” has been submitted to Physical Review A from part of the work done for this thesis. vii Table of Contents 1. Introduction 1 2. Overview of MOT and Molasses a. MOT 2 b. Molasses 5 3. Tunable Laser Diodes 7 4. Saturated Absorption Spectroscopy 13 5. Hardware a. Vacuum System 18 b. Electronics 19 c. Magnetic Coils 21 d. Optics 21 6. Trap Characterization 23 7. Intensity Correlations and Spatial Coherence 25 8. Radiation Trapping Theory 31 9. Experiment 36 10. Conclusion 40 11. References 41 12. Appendix A 42 13. Appendix B 48 viii List of Figures Figure Page Diagram of ball with trapping laser beams 2 Hyperfine energy levels of Rb 3 Splitting of J levels by magnetic gradient 4 Picture of laser diode set-up 8 Schematic of laser diode set-up 10 Schematic of saturated absorption set-up 14 Picture of saturated absorption set-up 16 Pictures of hyperfine spectra on oscilloscope 17 Diagram of ball and detector for theoretical calculation of spatial coherence 26 Diagram of image of atoms in image plane of detector 30 Plot of spatial coherence factor S 30 Theoretical plot of intensity correlation function 35 Picture of intensity correlation detection system 36 Schematic of intensity correlation detection system 37 Experimental plot of intensity correlation function 39 Sample data set 44 1 Introduction Cold atoms have become a topic of considerable interest in recent years. With Nobel prizes awarded in the field in 2001 and 1997, interest in laser cooling and trapping has only been growing. Cold atoms have been used in clocks [1] and atom lasers [2], and Bose-Einstein condensates have become a very interesting area of study [3,4,5]. The Magneto-Optic Trap (MOT) is the workhorse of many systems involving cold atoms, and is the system that we have assembled in the last two and a half years. Basically, a MOT is the slowing and confinement of atoms using lasers and magnetic fields. This technique can result in cooling the atoms in the MOT to temperatures in the microKelvin range. The purpose of our recent work has been to study the effect of radiation trapping in optical molasses. Optical molasses is the effect occurring when the magnetic fields in a MOT are turned off and the atoms are slowed (but not confined) by the light beams used in the MOT. Radiation trapping, meanwhile, is an effect that occurs when photons emitted by atoms in the trap are absorbed by other atoms in the trap [6,7]. Hence, the radiation, in the form of photons, is confined to the trap. It is useful to study radiation trapping because it imposes a limit on how cold and dense the trap can be made. Our method for examining radiation trapping is to look at intensity correlations [8] of light scattered from the atoms. Because radiation trapping is a result of spontaneous emission, we expect that the effect of radiation trapping will result in less correlated data, as spontaneous emission is a random process. 2 Overview of MOT and Molasses y Fig. 1 Trapping Laser Beams from 6 Directions MOT The MOT is formed by directing laser beams into a gas of atoms (in our case, Rb) from three perpendicular directions and reflecting these beams back onto themselves, with the net result being six beams hitting the atoms from six directions (Fig. 1). The wavelength of these beams is slightly red detuned from the 780 nm atomic resonance so that atoms with velocities directed opposite to a given beam are Doppler-shifted into resonance. Thus, only atoms traveling against a beam “see” the light and absorb photons. Now, when an atom absorbs a photon from one of these beams, it receives some momentum in the direction of the beam through conservation of momentum. Of course, it will re-emit this photon at some later time, but because spontaneous emission occurs in a 3 random direction, the average momentum from spontaneous emission is 0. The end result of this process is that atoms moving into the beam are slowed as they absorb photons, an effect known as optical molasses. We find that Rubidium is an excellent atom to use for this type of experiment due to its relatively simple hyperfine structure and the ease with which we can find diode lasers of the correct wavelength. Trapping Transition Repumper Fig. 2 Diagram of Rb energy levels with hyperfine splitting and labeling of trapping and repumping transitions Two optical frequencies are actually required to trap atoms, which is, in our case, 2 provide by two separate lasers. Our main trap laser pumps from the 5 S1/2, F = 3 ground 2 state to the 5 P3/2, F′ = 4 excited state. However, our laser off-resonantly pumps some of 4 the atoms into the other hyperfine levels in the 3/2 excited state. These levels allow a transition down to the F = 2 ground state, where the atoms no longer interact with the trapping laser, and eject from the trap. Thus, a second laser which pumps from the F = 2 ground state back to the F′ = 3 excited state is required, as shown in Fig. 2. We refer to this second laser as the “repumping” laser. If we did not have this second laser, all of the atoms would end up in the F = 2 ground state within a millisecond. E J = 1 1 State 0 -1 νlaser J = 0 State x Fig. 3 Splitting of excited state electronic energy levels by a magnetic gradient Next, we turn on a magnetic gradient, which has the effect of imparting a position dependent shift to the energy levels of the atom. J, the interaction of the electronic orbital angular momentum and the nuclear spin, reacts to a magnetic field by splitting the excited state energy levels into three quantized values depending on the value of the 5 magnetic field. With the energy levels shifted slightly by a magnetic gradient, such as in Fig. 3 we find that the atom has a greater probability of absorbing the laser light when it is polarized in a certain way, either σ+ or σ- circular polarization. Because the –1 J state is more easily pumped by σ- light and the +1 J state is more easily pumped by σ+ light, if the set-up was as shown in Fig. 3, we would adjust the polarization of the light to be σ- from the right and σ+ from the left. This provides the position-dependent force to trap the atoms. The atoms are more likely to absorb light if they stray from the center of the chamber, the place of 0 magnetic field. Without the magnetic field gradient, the atoms are slowed, but have no preferred gathering place. We are using the MOT to measure the effect of radiation trapping using intensity correlations of light scattered from the trap. The intensity correlation function is the probability of observing a pair of emitted photons separated by a certain time delay. Both the concepts of intensity correlations and radiation trapping are explained in detail after the explanation of the construction of the MOT. Optical Molasses As discussed above, a magnetic gradient is necessary to supply a position dependent force on the atoms, which pushes them towards the center of the chamber to create a trap.
Details
-
File Typepdf
-
Upload Time-
-
Content LanguagesEnglish
-
Upload UserAnonymous/Not logged-in
-
File Pages59 Page
-
File Size-