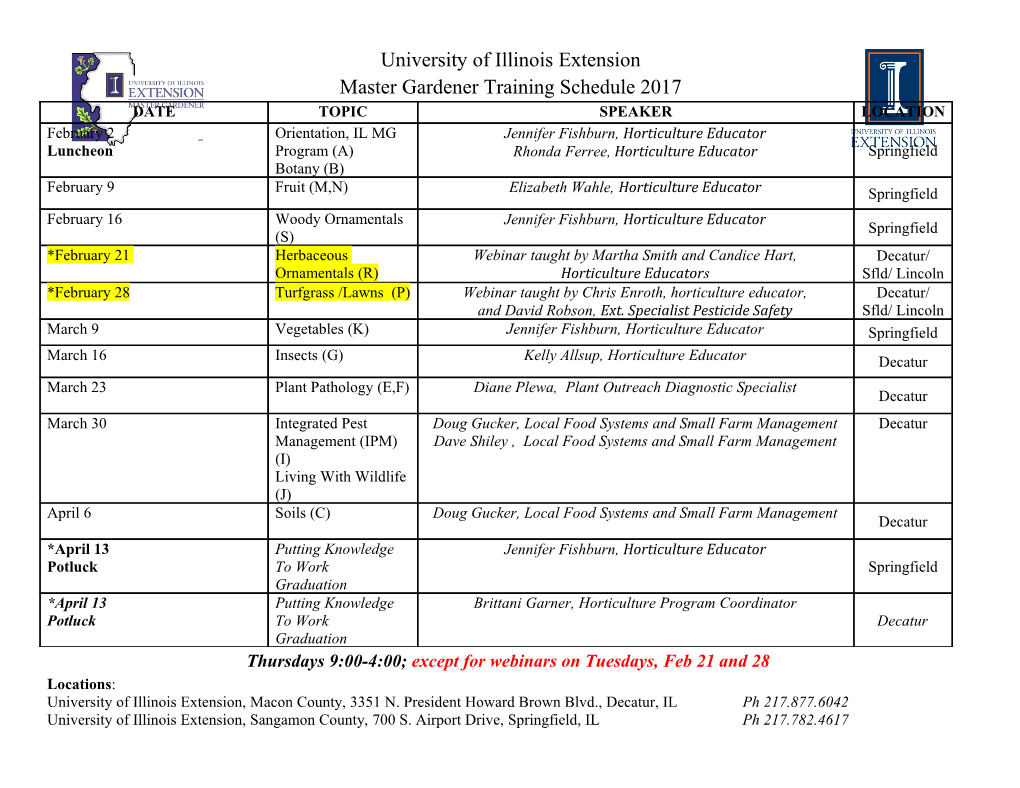
Air influence on the temperatures inside a concrete bridge box section Cristina Cachada, March of 2010 Summary The temperature on a bridge is a complex phenomena caused by several sources and it is quite difficult to forecast. Its effects cannot be ignored and during the last decades various studies have been made in this field. In order to reduce the complexity of these investigations, some simplifications, which do not entail large risks, are admitted making the problem easier to analyse. The main objective of this thesis is to analyse the air influence on the temperatures inside a concrete bridge box section . Usually this ‘detail’ is ignored or simplified by the investigators. With the work developed and presented in this thesis it is possible to understand the dimension of the error introduced in the studies every time this simplification is introduced. To fulfil this purpose, two finite element models were developed in order to compare with experimental values. The experimental values were collected using the instrumentation installed on the new Svinesund Bridge in Sweden. In both the models (A and B) several thermal sources were introduced affecting the cross section from the outside such as air temperature, solar radiation and the night radiance. The difference between the two models is that in the first model (A) the air inside the box cross-section is taken into account and in the second model (B) that air is ignored. The quality of the fit of the values obtained using the models A and B with the experimental values from Svinesund allow to take the conclusions of this work. The difference between the models does not indicate that changes in this detail must be included in the future thermal studies. Introduction Through the bridge design, the thermal effects that will affect the structure during its construction and its lifetime must be considered. The lack of constructive details to prevent the thermal effects, like the application of expansion joints, sliding or flexible bearings, and the adoption of flexible substructures, may culminate in some problematic situations [1]. Not all the consequences of the thermal action are catastrophic. Most current situations are concrete cracking which can be a matter of concern if it exceeds the regulation limits or there is the possibility of ultimate failure condition to be reached by reinforcement steel [2]. Most studies on this subject require research work over an extended period of time. The main objective of their authors usually is to find an efficient method to include the thermal action on bridge design. In order to analyze the influence of the air temperature inside the bridge cross section, a model with two versions (one that considers the air inside the section and one that does not) was created, and a comparison between the model’s results and measured values was made. Svinesund Bridge concrete arch was used to make the measurements. Heat Transmission Heat transmission is the propagation of heat through a certain substance and it can occur by several ways being the conduction, convection and solar and thermal radiation, the most significant for this work. Conduction is the phenomena of heat transference inside and between solids and it occurs due to temperature differences inside the body and in the surrounding environment [3]. Once, all the study is based on the arch cross section, it was used the Fourier’s heat flow equation adapted to 2D: S Sv Sv ̽ S/ Ɣ ͅ ʠS3v ƍ S4vʡ ƍ 3 ͋, where is the density of the fluid [kg/m ], ̽ is the specific heat of the concrete [J/(kg°C) or J/(kgK))] and ͅ 1 is the conductivity of the concrete [W/(m 2°C) or W/(m 2K)] [4]. The boundary conditions for this equation are S S 2 and where is the boundary input or loss per unit area [W/m ] and ͅ ʠS3 ͬ͢ ƍ S4 ͭ͢ʡ ƍ ͥ Ɣ 0 ͥ Ɣ ͥ. ƍ ͥ ƍ ͥ- ͥ , and represent energy transferred between the surface and the environment due to solar radiation, ͥ. ͥ ͥ- 2 convection and thermal radiation respectively [W/m ], finally ͬ͢ and ͭ͢ are the director cosines of the unit outward vector normal to the boundary surface. Convection is a way of heat transfer associated to heat exchanges inside a fluid or between a fluid and a solid surface. It occurs due to the movement of the fluid particles [3]. The air is the most influent fluid in convection actions and it is the only one considered in this study. The convection is included in the boundary conditions presented in the previous chapter and it can be represented by Newton’s cooling law: ͥ͗ Ɣ , w here is the energy transferred between the surface and the environment due to convection ͗͜ʚ͎ͧ Ǝ ͎͕ͦ͝ ʛ ͥ [W/m 2], is the temperature of the surface [°C or K], is the air temperature [°C or K] and is the ͎. ͎$- ͜ convection heat transfer coefficient [W/(m 2°C) or W/(m 2K)] [5]. This coefficient was calculated using ͯͥ ͤ,ͫͬ ͯͥ is the wind’s Nevander and Elmarsson theory: ͜ Ɣ 6 ƍ 4ͪ ʚͪ ƙ 5ͧ͡ ʛ and ͜ Ɣ 7,4ͪ ʚͪ Ƙ 5ͧ͡ ʛ where ͪ velocity [6]. The radiative heat transfer can then be described by Stefan-Boltzmann’s law as 4 4 ͥͦ Ɣ ʚ͎ͧ Ǝ ͎͕ͦ͝ ʛ is the thermal emissivity, is the Stefan-Boltzmann’s constant valuing 5,729 x 10 -8Wm -2°K -4, is where ͎. the absolute temperature of the surface [K] and is the absolute air temperature [K] [5]. ͎$- The solar radiation that reaches the earth’s surface is called global or total radiation. It is composed essentially by three parts: the beam radiation, the sky diffuse radiation and the contribution from surrounding reflected radiation (ground radiation). The beam radiation is also called direct radiation and can be described as the component that reaches the surface in a unidirectional way without any scattering [7]. The indirect radiation is also known as diffuse radiation is the result of successive reflections and refractions in the atmosphere [3]. In clear days the diffuse radiation has a small contribution, however, in cloudy days it can be the only component reaching a surface [2]. The determination of some components of the radiation such as the global or diffuse radiations can be done using electronic devices. The surrounding reflected radiation depends on the ground cover. In fact, the percentage of total radiation that is reflected by the ground is a function of the characteristics of the nearby elements such as the type of vegetation or the existence of water. The rate of heat absorbed by a surface due to short wave solar radiation can be represented by where is the solar energy absorbed by a surface (W/m 2), is the absorption coefficient of the ͥͧ Ɣ ̓ ͥ. 2 surface material ̓ is the solar radiation (W/m ) [8]. Box air temperature studies Some perspectives and studies from different authors about the influence of the air temperature inside the concrete bridge cross sections helped giving ideas to build the model. For example, Silveira refers inside the box the wind’s velocity is almost null and its value can be taken as zero [2]. 2 Branco and Mendes refer the importance of considering the heat exchange in the internal surfaces, by convection and irradiation. They say this temperature exchange is equal to the increase of energy of the box air volume. According to Elbadry and Ghali the air temperature inside the box section depends on the time variation of the temperature field on the inside surface of the box [8]. Svinesund Bridge [9] One way of understand the importance of considering the interior air temperature inside a co ncrete bridge cross section is by comparing t he measurements from a bridge’s cross section with the obtained values using a computer model. However the equipments and the monitoring to make studies are quite expensive and an alternative solution was explor ed. Using some of the instrumentation installed by KTH (Royal Institute of Technology) and installing some extra equipment, the study in a cross section of the arch of Svinesund Bridge was made. The Svinesund Bridge, Figure 1 , has an important role in the frontier between Sweden and Norway and it makes part of the motorway that links Goteborg to Oslo. The Svinesund Bridge is the world’s largest bridge with just a sin gle arch in the middle. The bridge is composed by two approach bridges and one central arch section. The arch span width is 247,3m and the entire bridge has an overall length of 704m. The arch bridge is made of reinforced concrete. The crown of the arch is located 91m above the sea level and its section has 4,4m x 2,7m. The arch presents one radial shape with a 154,226m radius. Figure 1 - Svinesund Bridge The numerical Model The model represents the cross section S25 (Figure 2) placed at the top of the concrete arch of the Svinesund Bridge because this section was already equipped with some of the thermal transducers needed for this study. 3 Figure 2 - Real dimensions of the cross section S25 (provided by KTH) The geometry used in the computational program was obtained using two rectangles: one defining the outside border and the other the inside hole. The positions of the temperature sensors inside the concrete walls were adapted to this geometry. Figure 3 - Geometry of the modelled section and positions of the temperature sensors Since the main objective of this work is to compare two models: one considering the air influence inside the box and other ignoring it, some actions that will be defined can only be applied to the first case.
Details
-
File Typepdf
-
Upload Time-
-
Content LanguagesEnglish
-
Upload UserAnonymous/Not logged-in
-
File Pages8 Page
-
File Size-