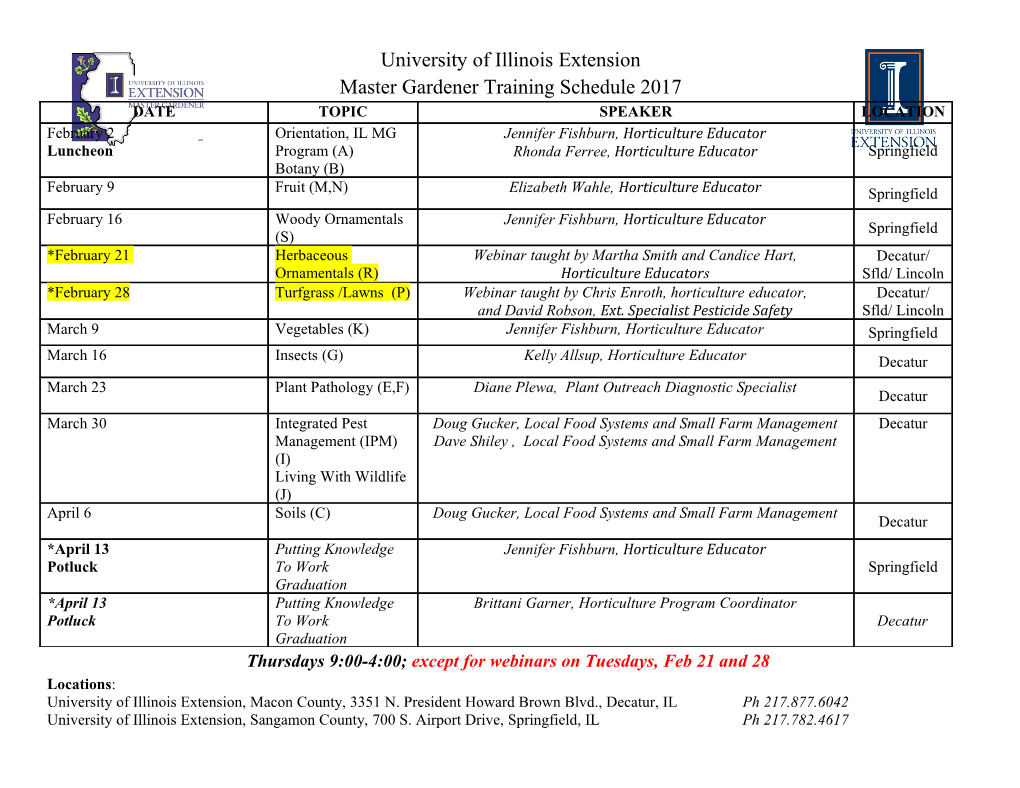
High-affinity single-domain binding proteins with a binary-code interface Akiko Koide, Ryan N. Gilbreth, Kaori Esaki, Valentina Tereshko, and Shohei Koide* Department of Biochemistry and Molecular Biology, University of Chicago, 929 East 57th Street, Chicago, IL 60637 Edited by David Baker, University of Washington, Seattle, WA, and approved March 1, 2007 (received for review January 7, 2007) High degrees of sequence and conformation complexity found in work, to establish the generalizability of the binary code natural protein interaction interfaces are generally considered concept, we set out to define its limitation by applying this essential for achieving tight and specific interactions. However, it approach to a small, single-domain scaffold. has been demonstrated that specific antibodies can be built by We have developed the 10th fibronectin type III domain of using an interface with a binary code consisting of only Tyr and Ser. human fibronectin (FNfn10) as the molecular scaffold for en- This surprising result might be attributed to yet undefined prop- gineering binding proteins (8, 9). FNfn10 is a small (Ϸ94 aa) erties of the antibody scaffold that uniquely enhance its capacity -sandwich protein with an overall fold similar to that of the Ig for target binding. In this work we tested the generality of the domain (9). We constructed combinatorial libraries by diversi- binary-code interface by engineering binding proteins based on a fying three loops (BC, DE, and FG) (Fig. 1A) located at one end single-domain scaffold. We show that Tyr/Ser binary-code inter- of FNfn10 and selected binders with desired characteristics. We faces consisting of only 15–20 positions within a fibronectin type have obtained such binding proteins, termed ‘‘monobodies,’’ III domain (FN3; 95 residues) are capable of producing specific with a Kd in the sub- to low-micromolar range from these binding proteins (termed ‘‘monobodies’’) with a low-nanomolar libraries (8, 9). Although monobodies can be considered anti- Kd. A 2.35-Å x-ray crystal structure of a monobody in complex with body mimics, both the size of the molecule and the number of its target, maltose-binding protein, and mutation analysis revealed surface loops available for diversification are considerably dominant contributions of Tyr residues to binding as well as smaller than those for the Fab scaffold. Thus, the monobody striking molecular mimicry of a maltose-binding protein substrate, system is a good platform for testing the minimalist limit of the -cyclodextrin, by the Tyr/Ser binary interface. This work suggests Y/S binary interface. that an interaction interface with low chemical diversity but with significant conformational diversity is generally sufficient for tight Results and specific molecular recognition, providing fundamental insights Engineering of Y/S Monobodies. We designed a Y/S binary com- into factors governing protein–protein interactions. binatorial library of monobody by introducing diversity at all positions in the three loops (BC, DE, and FG) (Fig. 1A) except 3D domain swapping ͉ antibody engineering ͉ combinatorial library ͉ for V27, where the V27S mutation significantly destabilized phage display ͉ sequence diversity the protein. Based on the studies of synthetic antibody libraries that showed the importance of loop length variation for rotein–protein interactions are of central importance in generating high-affinity binding (10), we varied the lengths of Pbiology. Studies of the protein–protein interaction have the three recognition loops in our library (6–10, 4–10, and demonstrated that, although the interfaces contain diverse 9–13 residues for the BC, DE, and FG loops, respectively). We ultimately constructed a single phage-display library contain- amino acid composition, there is significant bias in this compo- 10 sition toward specific residues (1). The observation of such bias ing 10 independent clones. This library size is comparable to within the antigen-binding sites of antibodies recently prompted the total number of possible sequences encoded by the design Ϸ ϫ 10 Sidhu and coworkers (2, 3) to explore the engineering of Fabs ( 2 10 ). from restricted combinatorial libraries in which positions in the We sorted the Y/S monobody library against three protein complementarity-determining regions are diversified to a com- targets, maltose-binding protein (MBP), human small ubiquitin- bination of as few as two amino acids. In the most surprising like modifier 4 (hSUMO4), and yeast small ubiquitin-like mod- example they used a binary combination of Ser and Tyr at a total ifier (ySUMO) (Fig. 1B). After three rounds of phage-display of 28–36 positions to successfully obtain specific Fabs with a library sorting, we transferred the enriched pools of monobody clones into the yeast-display format and performed one round of dissociation constant (Kd) of low nanomolar to low micromolar (2). Structural data showed a dominance of tyrosine in interface sorting. We were successful in obtaining binding clones to each contacts, and from these and other studies (1, 4–7) the particular of the targets. importance of Tyr as an interface-forming residue has emerged. The obtained Y/S monobodies (Fig. 1B) exhibited a clear and One might expect that the success of the Y/S binary Fabs distinct consensus sequence for each target. The selected clones may largely be due to the antibody-derived scaffold system, which has possibly evolved to acquire a uniquely high capacity Author contributions: A.K., R.N.G., and S.K. designed research; A.K., R.N.G., K.E., V.T., and for forming high-performance binding interfaces. Further- S.K. performed research; and A.K., R.N.G., V.T., and S.K. wrote the paper. more, the antigen-binding site of the antibody scaffold consists The authors declare no conflict of interest. of six complementarity-determining region loops allowing a This article is a PNAS Direct Submission. large number of residues to be involved in antigen binding. Abbreviations: CD, -cyclodextrin; FNfn10, the 10th fibronectin type III domain of human Thus, a fundamental question remains as to how general the fibronectin; hSUMO4, human small ubiquitin-like modifier 4; ySUMO, yeast small ubiquitin- Y/S binary interface is. It is not clear whether the binary code like modifier; MBP, maltose-binding protein; PDB, Protein Data Bank. approach would equally work for a smaller scaffold that Data deposition: The atomic coordinates and structure factors have been deposited in the contains fewer positions for diversification. One might expect Protein Data Bank, www.pdb.org (PDB ID code 2OBG). that the large number of Fab positions that can potentially *To whom correspondence should be addressed. E-mail: [email protected]. form a binding interface may create a ‘‘forgiving’’ environment This article contains supporting information online at www.pnas.org/cgi/content/full/ in which a combination of suboptimal amino acids in the 0700149104/DC1. interface can still generate tight and specific binders. In this © 2007 by The National Academy of Sciences of the USA 6632–6637 ͉ PNAS ͉ April 17, 2007 ͉ vol. 104 ͉ no. 16 www.pnas.org͞cgi͞doi͞10.1073͞pnas.0700149104 Downloaded by guest on September 23, 2021 Target clone # Kd (nM) BC-loop DE-loop FG-loop AB 2526272828a b c d 2930 52535455 757677787980818283848585a b BC MBP 74 (3) 81±13 S Y S S S VSGSKSYSYYYYYYS FG 76(2) 30± 3 SYSSY VY GSKS YSYYYYYYS N-term DE 79 (2) 89±32 S Y S S Y VSGSKSYSYYYYYYS 77 YSYYYS VS YYYS YSYYSYYSY 73 SYYYSY VS YYYS YSYYSSYYYY 712 SSYYY VS GSKS YYSYSSYSSYY 32 SSYYYYY VS GYSS YSYSSYYSYYY E 34 YSYYYYY VS GYSS YSYYSYYSYSY hSUMO4 33 (3) 7.0±0.1 S Y Y Y VSSYYSYYYYSYYYYYY B 36 SYYY VS SYYS YYYYSYYYSYYSY A G F C D 39 16±7 SYYY VS SYYS YYSSYYSSYYYSY 37 SSYY VS SYYS YYYYYSYYYSY 32 SSYY VS SYYY YYSYYSYYYYY 35 SSSYY VS YYYS YSSYYSYYSY 311 SYYYY VY YYYS YSSSYYYYY C-term 312 YYSSSYYYVS SYYS YSYYYYSYY ySUMO 53 (5) 7±2 S S S S VSSYYSYYYSYYYYYSY 55 SSSS VS GSKS YYYSYYYYYSY 56 5±3 SSSS VS SSYS YYYSYYYYYSY 59 SSSSSY VS SYYS YYSYYYYYYSY 57(2) 9±4 YYSYYSYSVS YYYS YSSYSSSSY Fig. 1. Amino acid sequences of Y/S monobodies. (A) A schematic drawing of the monobody scaffold. -Strands A–G and the three loops that are diversified in the library are indicated. (B) Affinity and amino acid sequences of Y/S monobodies that were selected from the initial library selection. The number of occurrences for clones that appeared more than once is indicated in parentheses. Kd values determined by using yeast surface display are also shown. The sequences for the three loops are shown, with the numbering of Main et al. (20). Tyr, Ser, and the other amino acids are shaded in yellow, red, and gray, respectively. were unique to their cognate target, and monobodies to different mechanism (Fig. 2B), and their dissociation constants (135 and targets show distinct loop length distributions (Fig. 1B). These 108 nM, respectively) were consistent with those determined results suggest that these monobodies are specific to their with yeast surface display (81 and 89 nM, respectively) (Fig. 1B). cognate targets, rather than nonspecific and ‘‘sticky.’’ The selected monobodies used all of the designed lengths of the BC and FG loops except for a 12-residue-long FG loop, suggesting that the BC and FG loops do not have a preferred 0.5 length. In contrast, the DE loop of all of the selected clones was 0.8 0.4 C A 0.3 four residues long, although its length was extensively diversified, 0.6 suggesting a strong preference imposed by the scaffold. Also, five 0.2 clones (ySUMO-55, MBP-74, MBP-76, MBP-79, and MBP-712) 0.4 0.1 BIOCHEMISTRY PE/FITC 0 contain the nonmutated DE loop (amino acid sequence GSKS) 74 0.2 76 (Fig.
Details
-
File Typepdf
-
Upload Time-
-
Content LanguagesEnglish
-
Upload UserAnonymous/Not logged-in
-
File Pages6 Page
-
File Size-