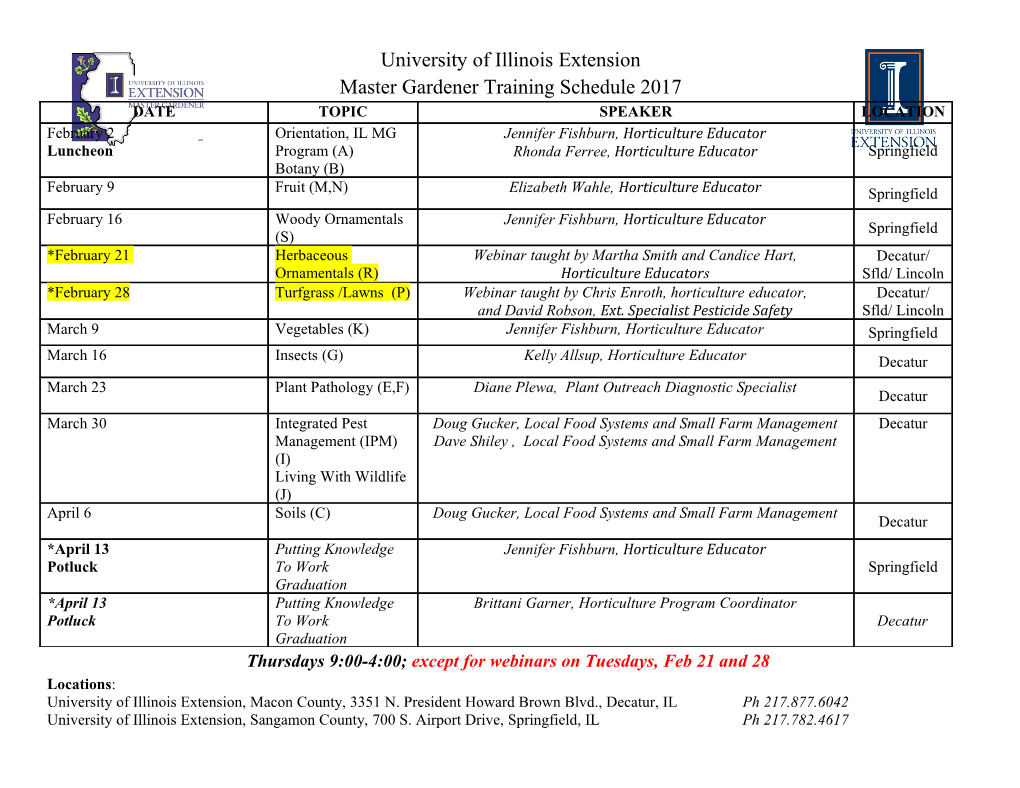
International Journal of Pure and Applied Mathematics ————————————————————————– Volume 56 No. 2 2009, 265-280 SIMULTANEOUS INTEGRAL REPRESENTABILITY BY INFINITELY SMOOTH KERNELS WITH APPLICATION TO INTEGRAL EQUATIONS Igor M. Novitskii Khabarovsk Division Institute for Applied Mathematics Far-Eastern Branch of the Russian Academy of Sciences 54, Dzerzhinskiy Street, Khabarovsk, 680 000, RUSSIA e-mail: [email protected] Abstract: In this note, we characterize families incorporating those bounded linear operators on a separable Hilbert space that can be simultaneously H transformed by the same unitary equivalence transformation into Carleman in- tegral operators on L2(R), whose kernels T : R2 C and Carleman functions → T (s, ): R L2(R) are infinitely smooth and vanish at infinity together with · → all partial and all strong derivatives, respectively. An explicit procedure for constructing the unitary operators, from onto L2(R), effecting such trans- H formations is also presented. As an application, we present a smooth version of Korotkov’s reduction method for general third-kind integral equations in L2(Y,µ), whose aim is to obtain an equivalent integral equation of either the first or the second kind in L2(R), with an infinitely smooth Hilbert-Schmidt or Carleman kernel, respectively. AMS Subject Classification: 47G10, 45P05, 47B33, 47B38, 47N20 Key Words: bounded Hilbert space operator, Hilbert-Schmidt operator, bounded integral linear operator, Carleman integral operator, characterization theorems for integral operators, linear integral equation of the third kind Received: September 7, 2009 c 2009 Academic Publications 266 Igor M. Novitskii 1. Introduction and the Main Result Throughout the note, is a complex, separable, infinite-dimensional Hilbert H space with norm and inner product , , and the symbols C, N, and · H · ·H Z, refer to the complex plane, the set of all positive integers, and the set of all integers, respectively. R( ) denotes the Banach algebra of all bounded linear H operators on . For an operator A in R( ), A∗ denotes the Hilbert space H H adjoint of A. Throughout let (Y,µ) denote a measure space Y equipped with a posi- tive, σ-finite, complete, separable, and not purely atomic, measure µ, and then let L2(Y,µ) denote the Hilbert space of (equivalence classes of) µ-measurable complex-valued functions on Y equipped with the inner product f, g L2(Y,µ) = 1/2 f(y)g(y) dµ(y) and the norm f 2 = f,f 2 . When µ is the Y L (Y,µ) L (Y,µ) Lebesgue measure on the real line R = ( , + ), we abbreviate L2(R,µ) R −∞ ∞ to L2, and dµ(y) to dy. An operator T R L2(Y,µ) is said to be integral if there is a µ µ- ∈ × measurable function T : Y Y C, a kernel, such that, for every f L2(Y,µ), × → ∈ (Tf)(x)= T (x,y)f(y) dµ(y) for µ-almost every x Y . ∈ ZY A kernel T on Y Y is said to be Carleman if T (x, ) L2(Y,µ) for µ-almost × · ∈ every fixed x in Y . Every Carleman kernel, T , induces a Carleman function t from Y to L2(Y,µ) by t(x)= T (x, ) for all x in Y for which T (x, ) L2(Y,µ). · · ∈ An integral operator with a kernel T is called Carleman if T is a Carleman kernel. Recall that a bounded linear operator U : L2(Y,µ) is unitary if U 2 H→ has range L (Y,µ) and Uf,Ug 2 = f, g for all f, g . L (Y,µ) H ∈H This note is written in the spirit of the pioneering work by Korotkov [5] on the simultaneous reducibility of families of linear operators to integral form by means of unitary equivalence transformations and its applications to integral equations. In that paper, Korotkov showed that if operators B R( ) (γ ) γ ∈ H ∈ G with an index set of arbitrary cardinality satisfy the condition B∗v , lim sup γ n H = 0 (1) n→∞ γ∈G where vn is an orthonormal sequence in , then there exists a unitary oper- { } 2 H −1 ator U : L (Y,µ) such that, for each γ , the operator Tγ = UBγU is H→ 2 ∈ G an integral operator on L (Y,µ), (Tγ f)(x) = Y Kγ(x,y)f(y) dµ(y) where the kernel K is Carleman. Motivated by this result, we consider in this note the γ R existence of a similar result when we specialize L2(Y,µ) to be L2 and require SIMULTANEOUS INTEGRAL REPRESENTABILITY BY... 267 each Carleman kernel Kγ to fulfill some additional analytic conditions that are to be technically useful in many applications, including the solving of integral equations. More specifically, we prove that when in (1) is at most countable, G the operators Bγ can simultaneously be made to have Carleman kernels that are SK∞ kernels in the sense of the following definition. Definition 1. A Carleman kernel T : R2 C is called an SK∞ kernel [9] → if it satisfies the two generally independent conditions: (i) the function T and all its partial derivatives on R2 of all orders are in C R2, C , dit (ii) the Carleman function t, t(s)= T (s, ), and its (strong) derivatives, i , · ds on R of all orders are in C R,L2 . An SK∞ kernel T is called a K∞ kernel [9] if the conjugate transpose ′ ′ function T (T (s,t)= T (t,s)) is also an SK∞ kernel, that is, if additionally ′ (iii) the Carleman function t′, t′(s) = T (s, ) = T ( ,s), and its (strong) ′ dit R R 2· · derivatives, dsi , on of all orders are in C ,L . Throughout this note, C(X,B), where B is a Banach space (with norm ), denotes the Banach space (with the norm f = sup f(x) ) ·B C(X,B) x∈X B of continuous B-valued functions defined on a locally compact space X and vanishing at infinity (that is, given any f C(X,B) and ε> 0, there exists a ∈ compact subset X(ε, f) X such that f(x) < ε whenever x X(ε, f)). ⊂ B ∈ We are now ready to state our main result. Theorem 2. Suppose that for a family = Br : r N R( ) there ∞ B { ∈ } ⊂ H exists an orthonormal sequence vn n=1 in such that ∗ { } H sup Br vn H 0 as n . (2) r∈N → →∞ Then there exists a unitary operator U : L2 such that, for each r N, the −1 H→ ∞ ∈ operator UBrU is a Carleman operator with an SK kernel. This result has recently been published without proof in [10, Theorem 2]. For the case in which is a singleton, its proof can be found in [9, Theorem B 1.4]. In [6, Section 3], there is a counter-example to show that the conclusion of Theorem 2 cannot in general be extended to operator families of more than countable cardinality. Section 2 of the present note is entirely devoted to proving Theorem 2. There we provide a direct method for constructing that unitary operator U : L2 whose existence the theorem asserts. The method uses no spectral H → 268 Igor M. Novitskii properties of the operators Br, other than their joint property imposed in (2), to determine the action of U by specifying two orthonormal bases, of and of H L2, one of which is meant to be the image by U of the other, the basis for L2 may be chosen to be an infinitely smooth wavelet basis. Section 3 of the present note deals with an application of Theorem 2 that is aimed at implanting the SK∞ kernels into integral equations. There we follow Korotkov’s method [5], applied to take into account Theorem 2, and reduce the general linear integral equation of the third kind in L2(Y,µ) to an equivalent integral equation either of the second kind (Theorem 3) or of the first kind (Theorem 4) in L2, with the kernel being the linear pencil of the SK∞ kernels or of the Hilbert-Schmidt K∞ kernels, respectively. 2. Proof of Theorem 2 The proof has two steps. The first step is to pick suitable orthonormal bases, f ,f ,f ,... for and u , u , u ,... for L2, and then to define a certain { 1 2 3 } H { 1 2 3 } unitary operator from onto L2 that carries f into u for each n, and is H n n suggested as U in the theorem. The second step is a straightforward verification that the constructed unitary operator is indeed as desired. Step 1. Assume that sup N B 1. This is a harmless assumption, r∈ r ≤ involving no loss of generality; just replace B with B > 1 by 1 B . Pick r r Br r ∞ ∞ · a subsequence, ek k=1, of the sequence vn n=1 of (2) such that { } { } 1 ∗ 4 M = sup Br ek H < (3) r∈N ∞ Xk (the sum notation k will always be used instead of the more detailed symbol ∞ ). For each r N, let k=1 P∈ 1 P S = B , Q = (1 E)S , J = S∗E, (4) r r · r r − r r r where E is the orthogonal projection of onto the closed linear span of the H ek’s, and note that ∗ Sr = Qr + Jr . (5) Assume, with no loss of generality, that dim(1 E) = . Let e⊥, e⊥, e⊥,... − H ∞ 1 2 3 be any orthonormal basis for the subspace (1 E) , and let f1,f2,f3,... be − H { ∞ } any basis for including all terms of the sequences e ∞ and e⊥ : H { k}k=1 k k=1 f ,f ,f ,... = e , e , e ,..
Details
-
File Typepdf
-
Upload Time-
-
Content LanguagesEnglish
-
Upload UserAnonymous/Not logged-in
-
File Pages16 Page
-
File Size-