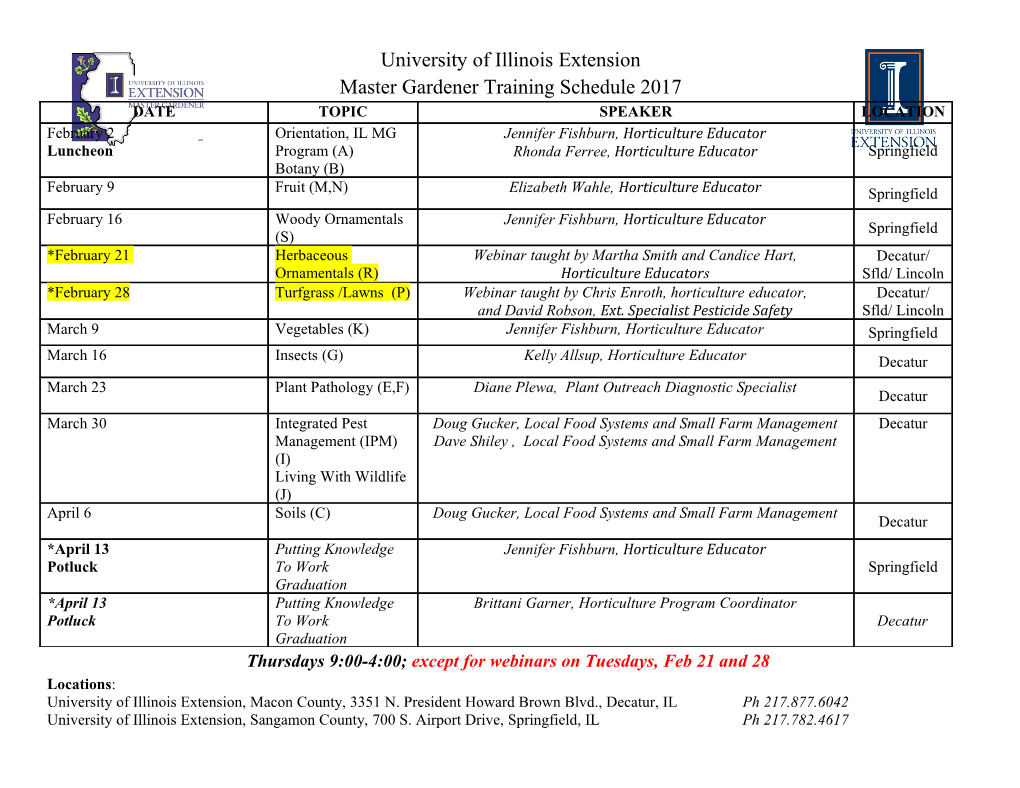
Viewpoint Cite This: ACS Catal. 2018, 8, 7898−7906 pubs.acs.org/acscatalysis Engineering Spatially Organized Multienzyme Assemblies for Complex Chemical Transformation Luke F. Bugada, Mason R. Smith, and Fei Wen* Department of Chemical Engineering, University of Michigan, Ann Arbor, Michigan 48109, United States − ■ INTRODUCTION enzymes.8 11 In recent years, researchers have drawn Over the past two decades, enzyme catalysis has emerged as an inspiration from natural enzyme colocalization and applied economical and environmentally friendly alternative to advances in protein engineering to create novel multienzyme conventional catalysis with numerous applications in the assemblies (MEAs) that provide extraordinary control over the pharmaceutical,1 food,2 and cosmetic3 industries. Among molecular ratio and spatial organization of the participating their advantages, many enzymes exhibit significantly greater enzymes. Several strategies have been employed to achieve fi control over these parameters including DNA scaffolds,12 activity, enantioselectivity, and speci city than their metal ff 13 14 counterparts,4 improving process efficiency and increasing protein sca olds, polymeric particle-based assemblies, metal−organic frameworks,15 and cross-linked enzyme aggre- product yields. In addition, enzymes tend to exhibit optimal 16 activities at relatively mild temperatures and pressures, gates. While structurally and mechanistically diverse, the ultimate goal of MEAs is to accelerate reaction rates and reducing energy consumption and thereby lowering operating ffi costs. The majority of industrial enzymes are used in single- increase reaction e ciency. step reactions; however, the potential applications of enzyme In our view, MEAs will become an increasingly attractive alternative to conventional catalytic processes for the catalysis can be greatly expanded by using multiple enzymes to fi catalyze complex chemical transformations. production of complex molecules in a variety of elds. Here Complex chemical transformations involve two or more we highlight promising advances in MEA engineering and enzyme-catalyzed reactions, which are related through summarize technical challenges that must be overcome for sequential, coupled, divergent, and/or convergent reaction these systems to be used in commercial production processes. − steps5 7 (Figure 1). Complex chemical transformations are common in biological processes ranging from protein synthesis ■ MULTIENZYME ASSEMBLY SYNTHESIS to cellular metabolism. In nature, microorganisms have evolved STRATEGIES features to maximize the catalytic efficiency of some complex MEAs can be synthesized using a variety of chemical and chemical transformations by colocalizing functionally related biomolecular strategies. While some of these chemistries have − been widely used for single enzyme immobilization,17 19 most strategies are enabled by recent advances in molecular − biology.20 22 Each strategy is associated with its own advantages and limitations (Figure 2), and the suitability of any one strategy to catalyze a complex chemical transformation is determined by the specific demands of that chemical reaction such as harsh reaction conditions, competing side reactions, enzyme activities, and whether the reaction takes place in vitro or intracellularly. Here, we will briefly introduce Downloaded via UNIV OF MICHIGAN ANN ARBOR on July 31, 2018 at 17:04:11 (UTC). common strategies for synthesizing MEAs to provide some See https://pubs.acs.org/sharingguidelines for options on how to legitimately share published articles. context for the sections below. Stochastic Colocalization. The simplest method to synthesize MEAs is the stochastic colocalization of enzymes, with the most common example being cross-linked enzyme aggregates (CLEAs, Figure 2a). CLEAs are formed by using salts, polymers, or organic solvents to precipitate enzymes from an aqueous buffer and cross-linking the resulting enzyme aggregates (5 to 50 μm)23 with a nonspecific fixative such as glutaraldehyde.16 CLEA synthesis is simple, amenable to rapid optimization, and applicable to a wide range of enzymes.24 As a Figure 1. Complex chemical transformations consist of two or more result, CLEAs and CLEA variants have been used as industrial 25 enzymatic reactions. (a) Enzymatic reactions can be linked to form a biocatalysts since the early 1990s. complex chemical transformation through four basic relationships: Another strategy for the stochastic colocalization of enzymes sequential, coupled, divergent, and convergent. (b) A hypothetical is immobilizing them on or encapsulating them within metal example of a three-enzyme complex chemical transformation that includes sequential and coupled reactions. E = Enzyme, S = Substrate, I = Intermediate, P = Product, C = Cofactor. Received: May 15, 2018 Published: July 17, 2018 © XXXX American Chemical Society 7898 DOI: 10.1021/acscatal.8b01883 ACS Catal. 2018, 8, 7898−7906 ACS Catalysis Viewpoint Figure 2. Overview of multienzyme assembly (MEA) synthesis strategies. (a) Cross-linked enzyme aggregates (CLEAs). (b) Metal−organic frameworks (MOFs). (c) Polymer assemblies. (d) Protein scaffolds. (e) DNA scaffolds. organic frameworks (MOFs, Figure 2b). MOFs are highly MEAs also provide limited control over the stoichiometric porous (typically 100 to 6000 m2/g),26 crystalline structures ratio of enzymes within the MEA. For example, it is more (0.1 to 50 μm) composed of inorganic nodes and organic difficult to encapsulate large enzymes than small enzymes,34 − linkers that can be tuned to adsorb specific molecules.26 28 In and not all enzymes will nonspecifically adsorb or bind to sites addition to providing a high-surface-area support, enzymes on a surface at the same rate. Nevertheless, stochastically organized on MOFs can benefit from the intrinsic affinity of assembled MEAs have proven to be useful for complex MOFs for a substrate and thereby concentrate the substrate chemical transformations involving the synthesis of pharma- near the MEAs.29 While the MOF MEAs are traditionally ceuticals, the production of bioethanol, and the manufacturing assembled through stochastic adsorption or encapsulation, a of food and textiles. recent study reported synthesis of a MOF that separated two Directed Assembly. In contrast to stochastic colocaliza- enzymes into distinct layers within the same MOF, offering tion, highly spatially organized MEAs can be synthesized by some spatial control over the enzyme assembly.15 directed assembly using high affinity, specific biomolecular Alternatively, enzymes can be randomly immobilized on the interactions involving proteins (Figure 2d) and nucleic acids surface of polymeric particles such as micelles30 (Figure 2c, (DNA or RNA) (Figure 2e). One of the most studied MEAs top) or polymersomes31 (Figure 2c, bottom). Polymersomes found in nature is the cellulosome,8 which is formed by the can also encapsulate enzymes within the polymer bilayer protein-scaffold directed assembly of cellulolytic enzymes. (Figure 2c, bottom),31 allowing additional enzymes to be Cellulosome assembly relies on the interaction between the incorporated into the MEA and avoiding immobilization (e.g., cohesin domains in the protein scaffold and the dockerin adsorption, cross-linking, etc.), which often reduces enzyme domains of enzymes. Cellulosome assembly has been applied activity. In order to allow reactants to access the encapsulated to a diverse range of biological problems35,36 and has inspired a enzymes, pores or channel proteins can be added during number of protein-scaffold directed MEA designs (Figure − bilayered polymersome synthesis.32 Another advantage of 2d).37 42 The cohesin−dockerin interaction is among the bilayered polymersomes is that their size can be easily tuned, strongest protein−protein interactions found in nature, with with diameters ranging from 100 to 1000 nm.33 This flexibility recorded binding affinities as low as 10 pM.43 Other protein provides a wide range of design possibilities and significant binding pairs with strong binding affinities, such as affibodies ∼ 44 control over enzyme density within or on the surface of (antibody mimetics with Kd 50 to 900 nM) and binding ∼ spherical polymersomes. domains involved in cellular signal processing (Kd 100 to While the features of each particular strategy vary, the 10 000 nM)13,45,46 are also commonly used in protein-scaffold primary advantage of using stochastic assembly to synthesize directed MEAs with MEA sizes on the order of ∼5to50 MEAs is the simplicity of the process because no prior genetic nm.38,45 or chemical modificationsoftheenzymesareneeded. DNA-scaffold directed MEA synthesis is generally per- However, in addition to offering little to no control over the formed by genetically fusing each enzyme to a DNA binding − spatial organization of enzymes, stochastically assembled protein47 49 or using a cross-linker to conjugate amine groups 7899 DOI: 10.1021/acscatal.8b01883 ACS Catal. 2018, 8, 7898−7906 ACS Catalysis Viewpoint Scheme 1. Mevalonate Synthesis Pathway in the enzyme to a thiol group added to a short single-stranded 77-fold increase of the mevalonate yield compared to cells DNA sequence.12,50,51 This enables the enzymes to interact without the protein scaffold.13 Intracellular protein-scaffold with DNA scaffolds with defined sequences at nanomolar directed MEAs have similarly been shown to increase product affinities (Figure 2e).12,52,53 This interaction combined with yields in other studies;45,46 however, their application can be DNA origami techniques enables DNA
Details
-
File Typepdf
-
Upload Time-
-
Content LanguagesEnglish
-
Upload UserAnonymous/Not logged-in
-
File Pages9 Page
-
File Size-