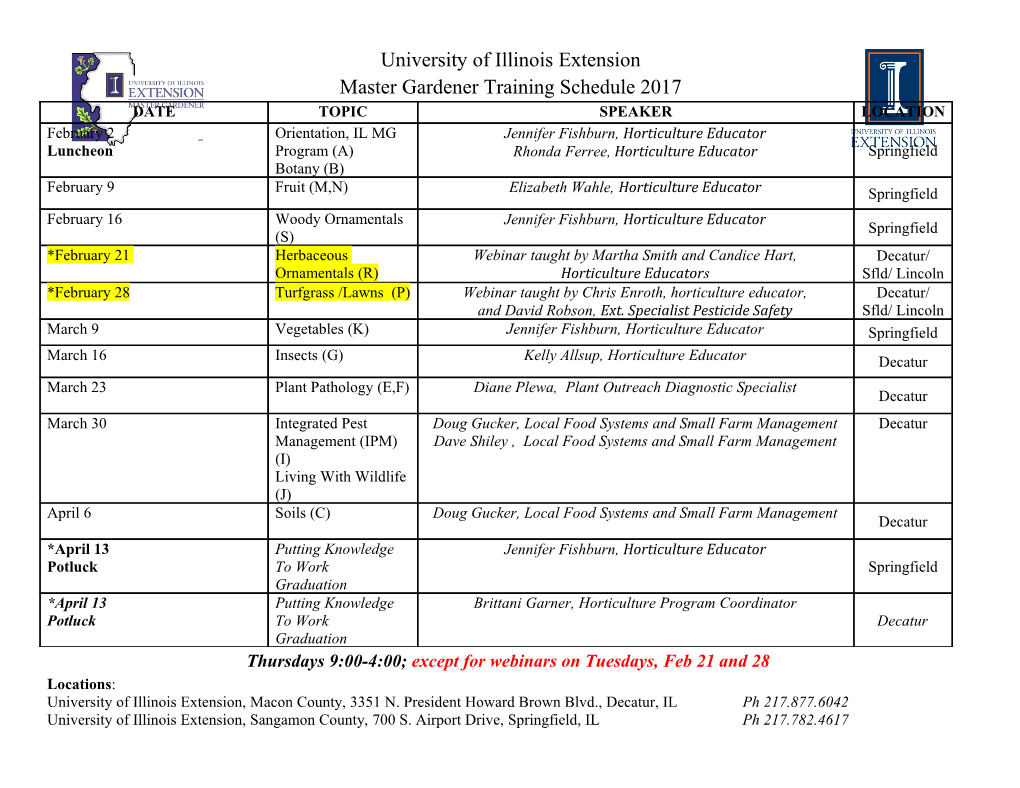
Fourier Analysis – IMA Summer Graduate Course on Harmonic Analysis and Applications at UMD, College Park John J. Benedetto Norbert Wiener Center Department of Mathematics University of Maryland, College Park http://www.math.umd.edu/˜jjb The Fourier transform Definition 1 The Fourier transform of f 2 Lm(R) is the function F defined as Z F(γ) = f (x)e−2πixγ dx; γ 2 Rb = R: R Notationally, we write the pairing between f and F as: ^f = F: 1 The space of Fourier transforms of Lm(R) functions is 1 ^ A(Rb) = fF : Rb ! C : 9 f 2 Lm(R) such that f = Fg: OPEN PROBLEM Give an intrinsic characterization of A(Rb): The Fourier transform inversion formula 1 Let f 2 Lm(R). The Fourier transform inversion formula is Z f (x) = F(γ)e2πixγ dγ: Rb Fˇ = f denotes this inversion. There is a formal intuitive derivation of the Fourier transform inversion formula using a form of the uncertainty principle. JB-HAA Jordan pointwise inversion formula Theorem 1 Let f 2 Lm(R). Assume that f 2 BV ([x0 − , x0 + ]), for some x0 2 R and > 0. Then, f (x +) + f (x −) Z M 0 0 = lim ^f (γ)e2πixγ dγ: 2 M!1 −M Example 1 ^ 1 f 2 Lm(R) does not imply f 2 Lm(Rb). In fact, if f (x) = H(x)e−2πirx ; where r > 0 and H is the Heaviside function, i.e., H = 1[0;1); then 1 ^f (γ) = 2= L1 (Rb): 2π(r + iγ) m Algebraic properties of Fourier transforms Theorem 1 a. Let f1; f2 2 Lm(R), and assume c1; c2 2 C. Then, ^ ^ 8 γ 2 Rb; (c1f1 + c2f2)^(γ) = c1f1(γ) + c2f2(γ): 1 ^ 1 b. Let f 2 Lm(R) and assume F = f 2 Lm(Rb). Then, 8 x 2 R; Fb(x) = f (−x): 1 c. Let f 2 Lm(R). Then, 8 γ 2 Rb; bf (γ) = ^f (γ): Translation and dilation For a fixed γ 2 R; we set 2πixγ eγ (x) = e : For t 2 R and f : R ! C, the translation operator, τt ; is defined as τt (f )(x) = f (x − t); and, for a fixed λ 2 R n f0g and for a given function f : R ! C, the dilation operator is defined by the dilation formula, fλ(x) = λf (λx): Example Dilation and the Poisson function If f (x) = e−2πrjxj, r > 0, then ^ 1 1 1 1 f (γ) = P1=r (γ) = 2 L (Rb); r r π(1 + γ2=r 2) m where P(γ) = 1=(π(1 + γ2)) is the Poisson function. Geometric properties of Fourier transforms Theorem 1 Let f 2 Lm(R), and fix t 2 R, ξ 2 Rb, and λ 2 Rb n f0g. Then, a. ^ (eξf )^(γ) = τξ(f )(γ); b. ^ (τt (f ))^(γ) = e−t (γ)f (γ); c. λ γ (f )^(γ) = ^f ( ): λ jλj λ Rotations play a major role in Fourier analysis on Rd : Formal properties of the Fourier transform Theorem a. f is real if and only if F(γ) = F(−γ): In this case, Z Z F(γ) = f (x) cos(2πxγ) dx − i f (x) sin(2πxγ) dx; R R Z 1 f (x) = 2 Re F(γ)e2πixγ dγ: 0 b. f is real and even if and only if F is real and even,. In this case, Z 1 Z 1 F(γ) = 2 f (x) cos(2πxγ) dx; f (x) = 2 F(γ) cos(2πxγ) dγ: 0 0 c. f is real and odd if and only if F is odd and imaginary. In this case, Z 1 Z 1 F(γ) = −2 i f (x) sin(2πxγ) dx; f (x) = 2i F(γ) sin(2πxγ) dγ: 0 0 Fourier transform of the Gaussian Example 2 Let f (x) = e−πrx , r > 0. We could calculate ^f by means of contour integrals, but we choose Feller’s real approach. Z 2 (^f )0(γ) = −2πi te−πrx e−2πixγ dx: R Noting that d 2 2 (e−πrx ) = −2πrx e−πrx ; dx integration by parts gives Z −1 2 −2πγ (^f )0(ξ) = −2πi (e−πrx )0e−2πixξ dx = ^f (γ): 2πr r ^ 0 2πγ Thus, f is a solution of F (γ) = − r F(γ); and so 2 ^f (γ) = F(γ) = Ce−πγ =r : Fourier transform of the Gaussian, continued Example Taking γ = 0 and using the definition of the Fourier transform, R −πrx 2 C = R e dx: In order to calculate C we first evaluate R 1 −u2 a = 0 e du. 1 1 1 1 Z 2 Z 2 Z Z 2 2 a2 = e−s ds e−t dt = e−(s +t ) dsdt 0 0 0 0 π=2 1 1 Z Z 2 π Z π = e−r rdrdθ = e−u du = : 0 0 4 0 4 R −u2 p Thus, R e du = π: Consequently, Z 2 1 Z 2 1 C = e−πrx dt = p e−u du = p : R πr R r 2 Therefore, ^f (γ) = p1 e−πγ =r : r Analytic properties of Fourier transforms Theorem 1 Let f 2 Lm(R). ^ a. 8 γ 2 Rb, jf (γ)j ≤ kf k1. b. 8 > 0; 9 δ > 0 such that 8 γ and 8 ζ, for which jζj < δ, we have j^f (γ + ζ) − ^f (γ)j < , i.e., ^f is uniformly continuous. Proof. ^ ^ R −2πixζ b. Note that jf (γ + ζ) − f (γ)j ≤ R jf (x)j e − 1 dx: −2πixζ Let gζ (x) = jf (x)j e − 1 . Since limζ!0 gζ (x) = 0 for all x 2 R, and since jgζ (x)j ≤ 2jf (x)j, LDC implies Z lim gζ (x) dx = 0; independent of γ: ζ!0 R Thus, ^ ^ 8 > 0; 9ζ0 > 0; 8 ζ 2 (−ζ0; ζ0) and 8 γ 2 Rb; jf (γ + ζ) − f (γ)j < . This is the desired uniform continuity. Analytic properties of Fourier transforms, continued 1 The following result for Lm(R) has essentially the same proof as the 1 Riemann-Lebesgue lemma for Lm(T), see ahead. Theorem 1 Riemann–Lebesgue lemma. Assume f 2 Lm(R). Then, lim ^f (γ) = 0: jγj!1 Example C0(Rb) n A(Rb) 6= ;. Define ( 1 log(γ) ; if γ > e; F(γ) = γ e ; if 0 ≤ γ ≤ e; on [0; 1) and as −F(−γ) on (−∞; 0]. Also, A(Rb) is a set of first category in C0(Rb). Even more, a Baire category argument can also be used to show the existence of F 2 Cc(Rb) for which F 2= A(Rb). JB-HAA. Analytic properties of Fourier transforms, continued Theorem Differentiation of Fourier transforms. a. Assume that f (n), n ≥ 1, exists everywhere and that f (±∞) = ::: = f (n−1)(±∞) = 0: Then, (f (n))^(γ) = (2πiγ)n^f (γ): n 1 b. Assume that x f (x) 2 Lm(R), for some n ≥ 1. Then, k 1 ^ 0 ^ (n) x f (x) 2 Lm(R), k = 1;:::; n − 1, (f ) ;:::; (f ) exist everywhere, and 8 k = 0;:::; n; (−2πi·)k f (·) ^(γ) = ^f (k)(γ): Smooth function and fast Fourier decay, and vice-versa. Absolute continuity has spectacular and essential role. JB-HAA Convolution and Fourier transforms 1 The convolution f ∗ g of f ; g 2 Lm(R) is Z Z f ∗ g(x) = f (t)g(x − t) dt = f (x − t)g(t) dt: R R Theorem 1 1 Let f ; g 2 Lm(R). Then, f ∗ g 2 Lm(R) and (f ∗ g)^(γ) = ^f (γ)g^(γ): 1 For the proof, f ∗ g 2 Lm(R) and Fubini–Tonelli give Z Z (f ∗ g)^(γ) = f (x − t)g(t)e−2πi(x−t)γ e−2πitγ dt dx R R Z Z = f (x − t)e−2πi(x−t)γ dx g(t)e−2πitγ dt R R Z = ^f (γ)g(t)e−2πitγ dt = ^f (γ)g^(γ): R This innocent proposition is actually a raison d’etreˆ for transform methods, generally, and for the Fourier transform. JB-HAA Approximate identity Definition 1 An approximate identity is a family fK(λ) : λ > 0g ⊆ Lm(R) of functions with the properties: R i. 8λ > 0; R K(λ)(x) dx = 1, ii. 9 M > 0 such that 8 λ > 0; kK(λ)k1 ≤ M, R iii. 8 δ > 0; limλ!1 jx|≥δ jK(λ)(x)j dx = 0, Theorem 1 R Let K 2 Lm(R) have the property that R K (x) dx = 1. Then, the 1 family, fKλ : Kλ(x) = λK (λx); λ > 0g ⊆ Lm(R), of dilations of K is an approximate identity. 1 Approximate identities in Lm(R) ⊆ Mb(R) approximate δ 2 Mb(R); where δ(f ) = f (0): δ is the multiplicative (under convolution) unit in the 1 Banach algebra Mb(R): The Banach algebra Lm(R) has no multiplicative unit. Examples of approximate identities Example a. The Fejer´ function W is 1 sin(x=2)2 W (x) = : 2π x=2 R W is non-negative and R W (x) dx = 1. Thus, the Fejer´ kernel 1 fWλ : λ > 0g ⊆ Lm(R) is an approximate identity. b. The Dirichlet function D is sin(x) D(x) = : πx R 1 Although R D(t) dt = 1, we have D 2= Lm(R). Thus, the Dirichlet kernel fDλ : λ > 0g is not an approximate identity. c. The Poisson kernel Pλ and the Gauss kernel Gλ; defined by 2 1 p1 −x P(x) = π(1+x 2) and G(x) = π e , are approximate identities.
Details
-
File Typepdf
-
Upload Time-
-
Content LanguagesEnglish
-
Upload UserAnonymous/Not logged-in
-
File Pages56 Page
-
File Size-