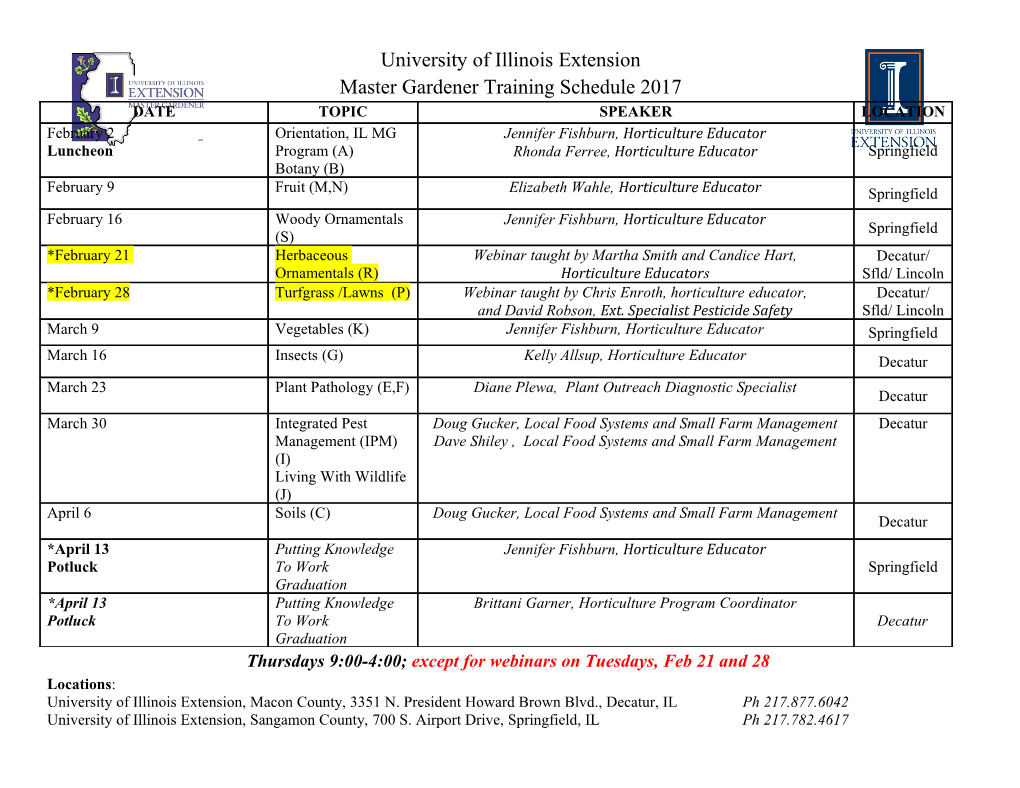
Sebastian Fähler and Kathrin Dörr, IFW Dresden Magnetic Shape Memory Alloys • Magnetically Induced Martensite (MIM) • Magnetically Induced Reorientation (MIR) • Requirements for actuation • “Exotic” materials www.adaptamat.com German Priority Program SPP 1239: “Modification of Microstructure and Shape of solid Materials by an external magnetic Field” www.MagneticShape.de Multiferroics magnetoelectric effect magnetic shape memory effect 2 Anisotropic magnetostriction Not important for Magnetic Shape Memory Alloys - Strain < 0.24 % + High frequency + Low magnetic field (spin-orbit Single ion effect coupling) – no collective phenomenon 3 Martensitic transformation T > T M: Austenite (high symmetry) T < T M : Martensite (low symmetry) No diffusion, reversible Twinned microstructure of martensite + Strain > 5% Thermal actuation + High forces ⇒ conventional shape memory effect - Low frequency 4 Prototype Ni-Mn-Ga, Shearing (1 1 0 ) Ni 2+x Mn 1-xGa L2 1 bcc - sheared Why are structures instable? 5 → Phonon spectra 7M Martensite 6 Martensitic transformation of magnets A. N. Vasil'ev, V. D. Ni 2.15 Mn 0.81 Fe 0.04 Ga Buchel'nikov, T. T / Takagi, V. V. K Khovailok, E. I. Estrin, 1 Physics Uspekhi 46(6) ~ Ferromagnetic (2003) 559 -588 d n Martensite a e it e s it n n e te t r s a u M A Non-magnetic Austenite Modification of structure and - High magnetic field shape by a magnetic field >> 1 T - Narrow temperature 7 regime Martensitic transformation of magnets A. N. Vasil'ev, V. D. Ni 2.15 Mn 0.81 Fe 0.04 Ga Buchel'nikov, T. T / Takagi, V. V. K Khovailok, E. I. Estrin, 1 Physics Uspekhi 46(6) ~ Ferromagnetic (2003) 559 -588 d n Martensite a e it e s it n n e te t r s a u M A Non-magnetic Austenite Modification of structure and - High magnetic field shape by a magnetic field >> 1 T - Narrow temperature 8 regime Magnetically Induced Martensite (MIM) Magnetic field favors ferromagnetic phase Clausius Clapeyron: dT ∆J = dH ∆S ∆J: magn. polarization difference in martensite and austenite state ∆S: entropy difference Magnetic actuation + Remote actuation Latent heat (magnetocaloric effect): here a problem 9 New Materials: Inverse Transformation Ni-Mn-In Magnetic field favors high temperature austenite because its ferromagnetism is stronger than that of martensite DSC: Magnetically weaker Martensite Magnetically stronger Austenite T. Krenke , M. Acet , E. F. Wassermann, X. Moya , L. Manosa, A. Shift of MS by -8 K/T Planes, Phys. Rev. B Large magnetocaloric effect 73 (17) (2006) 174413 10 Magnetically Induced Austenite (MIA) Magnetic field favors ferromagnetic phase Clausius Clapeyron: dT ∆J = dH ∆S ∆J: magn. polarization difference in martensite and austenite state ∆S: entropy difference Negative ∆ J → H stabilizes austenite 11 Magnetically Induced Austenite (MIA) Ni 45 Co 5Mn 36.7 In 13.3 FM Austenite R. Kainuma et al. Nature 439 (2006) 957 NM Martensite Hysteresis may inhibit + Strain ~ 3% reversibility ! Hysteresis losses? + No anisotropy needed 12 Rubber like behavior Ni-Mn-Ga, 7M At const T < T M F 3 2 HUT Finnland 1 HMI Berlin R. Schneider, HMI Berlin RecordedStress inMPa 0 0 2 4 6 8 10 12 Applied Strain in % Easy movement of twin boundaries - Little pinning of twin (~ MPa) boundaries at defects 13 Twin boundary movement A3: P. Entel, U. Duisburg-Essen Twin boudary Only highly symmetric twin boundaries are highly mobile But a collective movement would require to move 10 23 atoms simultaneously... 14 Microscopic view of twin boundary movement Dislocation (step + screw) as P. Müllner et al., JMMM 267 (2003) 325 elemental step of twin boundary movement „Intrinsic“ Peierls stress to S. Rajasekhara , P. J. Ferreira move Burgers vector ~ 10 -13 Pa Scripta Mat. 53 (2005) 817 15 Magnetically Induced Reorientation (MIR) Twin boundary movement No phase transition, affects only microstructure Requires: Non-cubic phase ++ Strain ≤ 10 % ! High magnetocrystalline aniosotropy + High frequency Easily movable twin boundary 16 Ferromagnet Rotation of magnetization must be avoided ⇒ high magnetocrystalline anisotropy needed 17 Domain and twin boundary dynamics Y.W. Lai, N. Scheerbaum , D. Hinz , O. Gutfleisch , R. Schaefer, L. Schultz, J. McCord, Appl . Phys. Lett. 90 (2007) 192504 TB 0 mT 200 mT H Magnetic field moves twin boundary instead of 18 magnetization rotation Integral measurement of strain and magnetization Ni-Mn-Ga 5 4 3 5M H H 3 4 2 2 5 1 HS O. Heczko, L. Straka, N. Lanska, K. Ullakko, J. Enkovaara, J. Appl. Phys. 5 4 3 1 91 (10) (2002) 8228 1 2 H o moderate switching field HS < 1 T 19 Setup of a linear actuator F F H=0 H1 H H F F H=0 20 2 3 Sebastian Fähler and Kathrin Dörr, IFW Dresden Magnetic Shape Memory Alloys • Magnetically Induced Martensite (MIM) • Magnetically Induced Reorientation (MIR) • Requirements for actuation • “Exotic” materials www.adaptamat.com German Priority Program SPP 1239: “Modification of Microstructure and Shape of solid Materials by an external magnetic Field” www.MagneticShape.de Beneficial conditions for MIR Intrinsic properties (composition, phase) • High martensitic transformation temperature ⇒ high application temperature • High magnetocrystalline anisotropy ⇒ avoids rotation of magnetization • High magnetization ⇒ high blocking stress ε =1− c • Large maximum strain 0 a Extrinsic properties (microstructure, texture) • High strain ε < ε 0 • Low switching field HS< H A • Easily moveable twin boundaries ⇒ rubber like behavior Aim: high strain in low magnetic fields 22 What is essential for the MSM effect? Not fulfilled for: Martensitic transformation Tb, Dy, Re Cu 2 Ferromagnetism Re Cu 2, La 2-xSr xCuO 4 High uniaxial magnetocrystalline Fe 70 Pd 30, Ni-Mn-In anisotropy High magnetostriction Ni-Mn-Ga Chemical ordering Fe 70 Pd 30 , Tb, Dy 23 Anisotropic magnetostriction Constrained 5M NiMnGa single crystals λ S = - 50 ppm O. Heczko , J. Mag. Mag. Mat. 290 -291 (2005) 846 Not appropriate to describe threshold like switching (Reorientation or Martensitic transformation) 24 Fe 70 Pd 30 Austenite: fcc Martensite: fct, c/a <1 two easy axis || a c a a R.D. James , M. Wuttig J. Cui , T.W. Shield, R.D. James , Phil. Mag. 77 (1998) 1273 Acta Mat. 52 (2004) 35 No uni axial anisotropy needed No chemical ordering 25 Tb 0.5 Dy 0.5 Cu 2 S. Raasch , et al. PRB 73 (2006) 64402 H no martensitic transformation orthorhombic (pseudohexagonal, 3 variants) .) .u /f B (µ M Canted magnetic order 26 1.5 % strain at 3.2 T by reorientation La 2-xSr xCuO 4 (LSCO) A. N. Lavrov , S. Komiya, Y. Ando, Nature 418 (2002) 385 A. N. Lavrov, Y. Ando, S. Komiya, I. Tsukada , Phys. Rev. Lett. 87 (2001) 17007 H = 14 T RT 1% strain 1 mm Orthorhombic, twinning in ab plane, b axis ( red domains ) aligns parallel to magnetic field Antiferromagntic , weak ferromagnetic moment 27 Dy, Tb Dy single crystal at 4 K 8% strain in Tb (40 T, 4K) S. Chikazumi et al. IEEE Trans. Mag. MAG -5(3) (1969) 265 J. J. Rhyne et al. J. Appl . Phys. 39 (2) (1968) 892 Pure elements H. H. Liebermann, C. D. Graham, Acta Met. 25 (7) (1977) 715 28 Ni-Mn-In Magnetic field favors high temperature austenite because its ferromagnetism is stronger than that of martensite H = 50 kOe DSC: Magnetically weaker Martensite Magnetically stronger Austenite T. Krenke , M. Acet , E. No significant magnetocrystalline anisotropy F. Wassermann, X. (cubic ferromagnet) Moya , L. Manosa, A. Planes, Phys. Rev. B 29 73 (17) (2006) 174413 Magnetic Shape Memory Alloys NiMnGa FePd Fe Pt Dy 3 Tb La 2-xSr xCuO 4 Re Cu MIR 2 Magnetic Martensite FMSMAMSMA Ferromagnet Ordering (SMA) MIM NiMnIn FeNiGa CoNiGa 30 Magnetic Shape Memory Alloys Magnetically Induced Martensite Magnetically Induced (MIM) Reorientation (MIR) Essential Martensitic transformation with Magnetocrystalline anisotropy large ∆J Easily movable twin boundaries Beneficial Low Hysteresis Ferromagnetism (High JS) Low ∆S Martensitic transformation Transformation around RT (rubber like behavior) Not needed Magnetocrystalline anisotropy Magnetostriction 31 32 Martensitic and Ferromagnetic Crystallographic variants Coupled by magneto- Magnetic domains Crystalline anisotropy H H F F Short axis aligned by stress Magnetization direction aligned by field Domain and variant movement → local mechanism 33 Martensitic and Ferromagnetic Crystallographic variants Coupled by magneto- Magnetic domains crystalline anisotropy H H F F Short axis aligned by stress Magnetization direction aligned by field Kerr microscopy: B1: J. McCord, 50 µm R. Schäfer, IFW Dresden Twin boundaries 90°and 180° (Variant boundaries, Domain boundaries grain boundaries) 34 Magnetic Shape Memory Alloys Magnetically Induced Martensite Magnetically Induced (MIM) Reorientation (MIR) + Little constrains on microstructure - Rubber like behavior needed + No magnetocrystalline anisotropy - High magnetocrystalline needed anisotropy Forces? - Low forces - High fields > 1 T + Moderate fields < 1 T - Works only at the vicinity of + Works below martensitic martensitic transformation transformation - Magnetocaloric effect inhibits high + High frequency (kHz) possible frequency 35.
Details
-
File Typepdf
-
Upload Time-
-
Content LanguagesEnglish
-
Upload UserAnonymous/Not logged-in
-
File Pages35 Page
-
File Size-