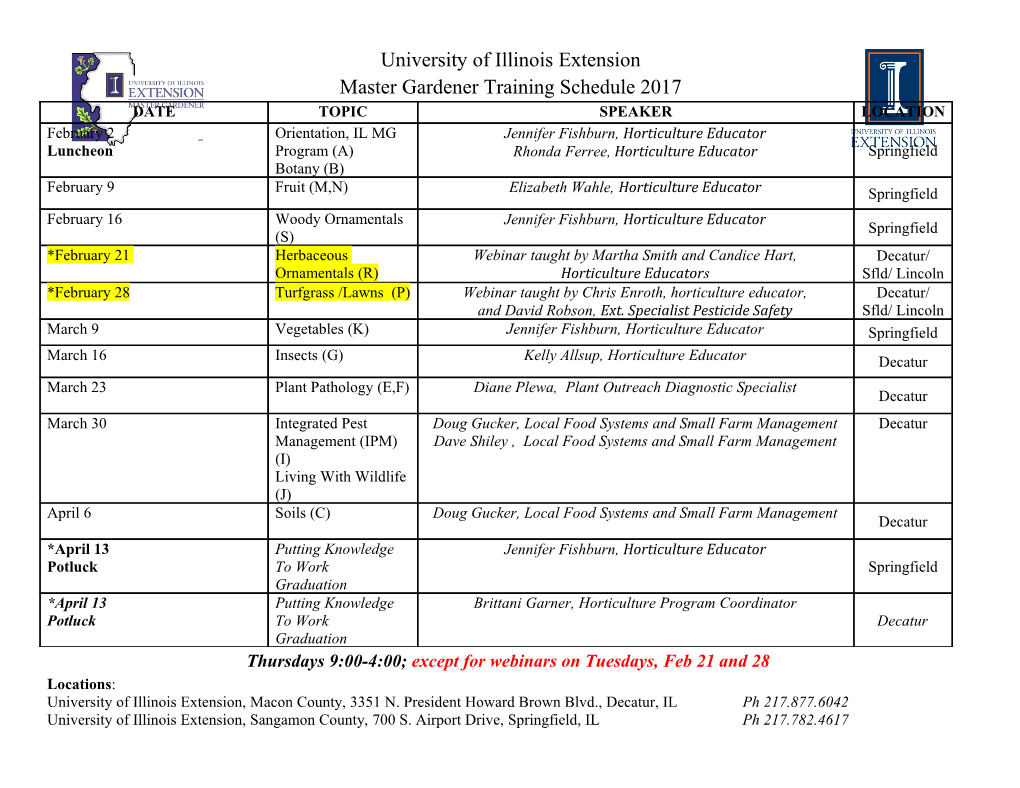
Bifacial Solar Cells: High Efficiency Design, Characterization, Modules and Applications Claudia Duran Dissertation der Universität Konstanz Tag der mündlichen Prüfung: 06. 09. 2012 1. Referent: Prof. Dr. E. Bucher 2. Referent: Prof. Dr. J. Boneberg Bifacial Solar Cells: High Efficiency Design, Characterization, Modules and Applications Dissertation zur Erlangung des akademischen Grades des Doktors der Naturwissenschaften (Dr. rer. nat) an der Mathematisch-Naturwissenschaftliche Sektion Fachbereich Physik vorgelegt von Claudia Duran 1. Referent: Prof. Dr. E. Bucher 2. Referent: Prof. Dr. J. Boneberg Tag der mündlichen Prüfung: 06. 09. 2012 Contents Introduction 1 Chapter I: Bifacial Solar Cells, high efficiency design 1.1 History of bifacial solar cells 4 1.1.1 Bifacial double junction cells 4 1.1.2 Bifacial back surface field solar cells 5 1.1.3 Bifacial solar cells with dielectric passivation 6 1.2 Monofacial vs. Bifacial 7 1.2.1 Saw damage removal and wafer cleaning 8 1.2.2 Boron diffusion and in-situ oxidation 9 1.2.3 Silicon Oxide (SiO 2) / Silicon Nitride (SiN x) stack layer 16 1.2.4 Etch back front side and Texturization 17 1.2.5 Phosphorus diffusion 19 1.2.6 Anti reflection coating SiN x front side 21 1.2.7 Screen printing 22 1.2.7.1 Front side 23 1.2.7.2 Rear side 24 1.2.8 Co firing and edges isolation 26 1.3 Solar cell results 28 1.3.1 Most relevant parameters 28 1.3.2 Solar cell losses 31 1.3.3 Initial I-V results over a batch of 50 solar cells 33 1.3.4 I-V results after sequence and steps optimization 34 1.3.5 Rear side change experiments 35 1.4 Light induced degradation (LID) 40 1.5 Further development: laser doping over boron diffusion 43 1.5.1 Introduction 43 1.5.2 Processing techniques 44 1.5.3 Solar cell process and results 46 1.5.4 Conclusion and outlook 51 Summary of Chapter I 52 Chapter II: Characterization of bifacial solar cells 2.1 I-V curve measurements 54 2.2 Spectral response measurements 57 2.3 Prerequisites for measurements 59 2.3.1 Transmittance of bifacial solar cells 59 2.3.2 Reflectance of different surfaces 60 2.4 Bifacial solar cell measurements 62 2.4.1 I-V for three different reflectance metal holders 62 2.4.2 Spectral response for two different chuck reflectances 63 2.4.3 I-V measurements for different reflectance metal holders 64 2.5 Rear side contribution 65 2.6 I-V both sides illumination 67 Summary of Chapter II 70 Chapter III: Bifacial PV modules 3.1 Introduction to PV modules 71 3.1.1 Front surface material 73 3.1.2 Encapsulant 73 3.1.3 “Identical” solar cells 74 3.1.4 Rear Surface 74 3.1.5 Frame 75 3.2 Bifacial PV mini-modules, different rear foils 76 3.2.1 QE measurements 76 3.2.2 I-V measurements 77 3.3 Double side transparent bifacial PV module 80 3.3.1 Construction 80 3.3.2 Packing density 80 3.3.3 Measurement of temperature coefficients 82 3.3.4 The data collection system for outdoor measurements 83 3.4 Measurement results 84 3.4.1 Outdoor measurements two different back surfaces 84 3.4.2 Different reflecting surfaces 86 3.4.3 Underlying area measurements 87 Summary of Chapter III 90 Chapter IV: Applications of bifacial solar cells and modules 4.1 Space applications 92 4.2 Solar trackers and concentrators 93 4.3 Terrestrial applications, albedo collection 95 4.4 BIPV 97 4.5 Vertical module, noise barriers 99 Summary of Chapter IV 101 Summary 102 Zusammenfassung 105 Acknowledgements Bibliography Introduction Economic growth is bound to energy consumption. Because anthropogenic emissions of carbon dioxide result primarily from the combustion of fossil fuels, energy consumption is at the center of the climate change debate. In December 2009, the fifteenth session of the Conference of Parties to the United Nations Framework Convention on Climate Change (COP-15) was held in Copenhagen, Denmark. After COP-15 there were no legally binding agreements to cut emissions, nevertheless the key developed and developing countries negotiated a Copenhagen Accord in its final session. Under the Accord, a process was established for countries to enter specific mitigation pledges by January 31, 2010. The emissions mitigation pledges submitted by countries pursuant to the Copenhagen Accord fall into two general categories: absolute reductions of greenhouse gas emissions and intensity reductions, independent of economic or material output [EIA]. The goals of the European Union for 2020 were set: “the renewable energies should have a 20% share of the total energy production and the overall CO 2 emission should be reduced by 20%” [ERE]. Renewable energies are nowadays the fastest-growing source of world energy. High projected oil prices, the concern about the environmental impacts of fossil fuel use and strong government incentives for increasing the use of renewable energy in many countries around the world have improved the prospects for renewable energy sources worldwide [EIA]. One way to achieve the proposed international goals is the use of photovoltaic solar energy, or more simply photovoltaics (PV). This is a method of generating electrical power by converting solar radiation into direct current electricity using semiconductors that exhibit the photovoltaic effect. Photovoltaic power generation employs solar panels composed of a number of solar cells containing a photovoltaic material. Materials presently used for photovoltaics include monocrystalline silicon, polycrystalline silicon, amorphous silicon, cadmium telluride, and copper indium gallium selenide/sulfide. “The demand for solar electric energy has grown in average 30% per annum over the past 20 years through a rapidly declining of costs for production and price of this energy. This decline in cost has been driven by economies of manufacturing scale, manufacturing technology improvements, and the increasing efficiency of solar cells” [SBZ]. 1 The growth rate of PV during 2011 reached almost 70%, an outstanding level among all renewable technologies [EPI]. “Bulk crystalline silicon dominates the current photovoltaic market, in part due to the standing of silicon in the integrated circuit market. In particular, silicon's band gap is slightly too low for an optimum solar cell and since silicon is an indirect gap material, it has a low absorption coefficient. While the low absorption coefficient can be overcome by light trapping, silicon is also difficult to grow into thin sheets. However, silicon's abundance, and its domination of the semiconductor manufacturing industry has made it difficult for other materials to compete” [PVE]. This work is based on crystalline silicon and more specifically on monocrystalline silicon. It explains the necessary processing steps to create a solar cell from a crystalline silicon substrate. These substrates called wafers are thin slices of semiconductor material formed of highly pure, nearly defect-free single crystal material. One process to form crystalline wafers is known as Czochralski growth. In this process, a cylindrical ingot of high purity monocrystalline silicon is formed. The technique uses a seed crystal which is pulled from a melt. The ingot is then sliced with a wafer saw and polished to form wafers. A silicon solar cell that is optimum in terms of light trapping and very good surface passivation is about 100 µm thick [PVE]. However, other thickness between 200 and 500 µm are typically used, in part for practical issues such as making and handling thin wafers, and partly for surface passivation reasons. Most of the used wafers in this work were about 200 µm with a base doping varying from 1-3 Ω·cm. Wafers are cleaned with diluted acids to remove unwanted particles, or repair damage caused during the sawing process [WIK]. They are further processed to become solar cells. Improving solar cell efficiencies while simultaneously lowering the cost per solar cell is an important challenging goal of the photovoltaic research and development. Possible ways to achieve this goal are: 1. Novel methods for the purification of crystalline silicon or the use of cheaper substrates. 2. Higher efficiencies using advanced processes, e. g. selective emitter structures. 3. Thinner wafers and higher power output using bifacial solar cell concepts. 4. Innovative solar cell concepts like IBC, Plasmon enhanced absorption, texturing, tandem hybrid systems. 2 This work is based on the concept presented in point number 3 and it has moreover used 1 and 2 concepts in different research stages. It began with the optimization of boron diffusion, necessary process to create this type of bifacial structure. After several attempts of process sequences and optimization of most fabrication steps, a final device was presented. It was published in 2010 by the author [CD2]. The device can be produced with good reproducibility and reliability of the results in a large quantity, within a small range of satisfactory efficiencies for the front side as well as for the rear side. Once this goal was achieved, we have tried to use a low quality material (Solar Grade Silicon) to show the performance of this concept. In a more advanced step we have developed the selective emitter on boron diffusion by means of laser doping. This new process was studied and published in 2009 by the author [CD1]. An important challenge about this type of silicon solar cells which is of special interest was the way these devices are measured. The peculiarity of the measurement problem is the fact that the daily standard industrial measurement systems influence the solar cell results. From this experimental discovery a thorough study was conducted, showing where and how big the influence of the measurement system is.
Details
-
File Typepdf
-
Upload Time-
-
Content LanguagesEnglish
-
Upload UserAnonymous/Not logged-in
-
File Pages130 Page
-
File Size-