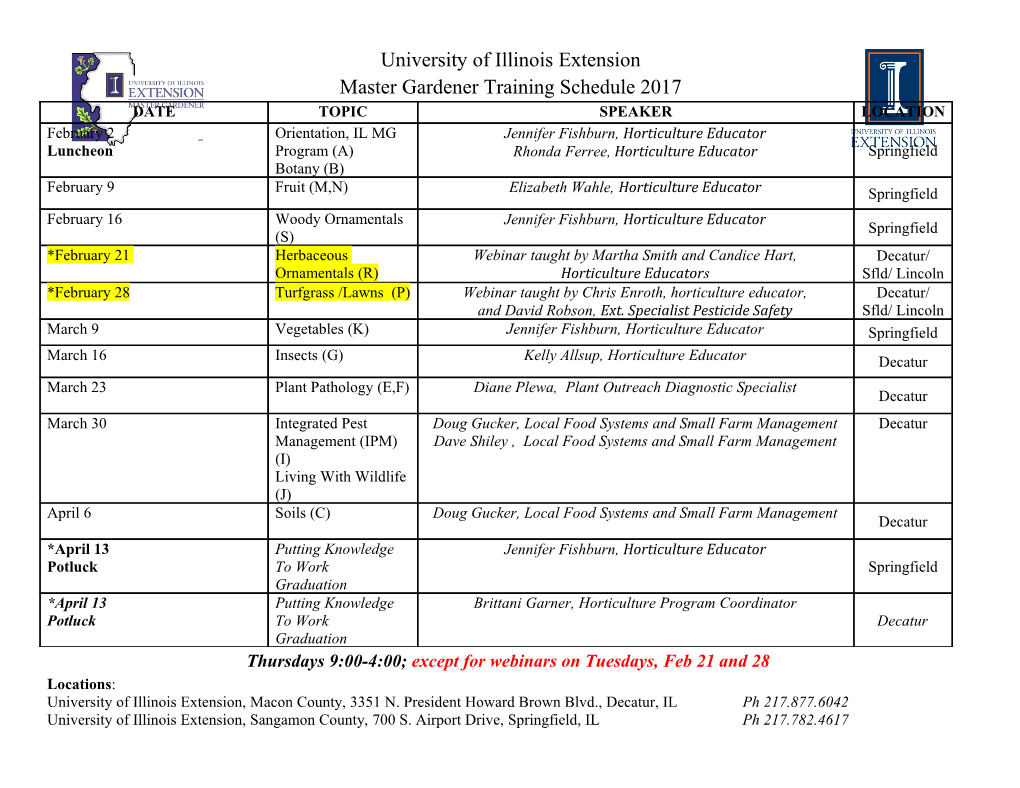
CHAPTER 10 CLINICAL USE OF CREATINE IN NEUROMUSCULAR AND NEUROMETABOLIC DISORDERS MARK A. TARNOPOLSKY Department of Pediatrics and Medicine (Neurology and Rehabilitation), Neuromuscular and Neurometabolic Clinic, Rm 2H26, McMaster University Medical Center, 1200 Main St. W., Hamilton, Ontario, Canada, L8N 3Z5 Abstract: Many of the neuromuscular (e.g., muscular dystrophy) and neurometabolic (e.g., mitochon- drial cytopathies) disorders share similar final common pathways of cellular dysfunction that may be favorably influenced by creatine monohydrate (CrM) supplementation. Studies using the mdx model of Duchenne muscular dystrophy have found evidence of enhanced mitochondrial function, reduced intra-cellular calcium and improved performance with CrM supplementation. Clinical trials in patients with Duchenne and Becker’s muscular dystrophy have shown improved function, fat-free mass, and some evidence of improved bone health with CrM supplementation. In contrast, the improvements in function in myotonic dystrophy and inherited neuropathies (e.g., Charcot-Marie-Tooth) have not been significant. Some studies in patients with mitochondrial cytopathies have shown improved muscle endurance and body composition, yet other studies did not find significant improvements in patients with mitochondrial cytopathy. Lower-dose CrM supplementation in patients with McArdle’s disease (myophosphorylase deficiency) improved exercise capacity, yet higher doses actually showed some indication of worsened function. Based upon known cellular pathologies, there are potential benefits from CrM supplementation in patients with steroid myopathy, inflammatory myopathy, myoadenylate deaminase deficiency, and fatty acid oxidation defects. Larger randomized control trials (RCT) using homogeneous patient groups and objective and clinically relevant outcome variables are needed to determine whether creatine supplementation will be of therapeutic benefit to patients with neuro- muscular or neurometabolic disorders. Given the relatively low prevalence of some of the neuromuscular and neurometabolic disorders, it will be necessary to use surrogate markers of potential clinical efficacy including markers of oxidative stress, cellular energy charge, and gene expression patterns 1. BACKGROUND OF THE CHAPTER The focus of this chapter will be on the potential clinical utility of CrM as an adjunctive therapy for the treatment of patients with neuromuscular and neurometabolic disorders. By definition, the neuromuscular disorders represent genetic and acquired conditions 183 G.S. Salomons and M. Wyss (eds.), Creatine and Creatine Kinase in Health and Disease, 183–204. © 2007 Springer. 184 Tarnopolsky that alter peripheral nerve and skeletal muscle function. Included in this category are also the neuromuscular junction defects such as myasthenia gravis which can be acquired (autoimmune) or congenital (due to defects in proteins involved in neuro- muscular transition). The peripheral neuropathies affect motor and/or sensory function and often lead to distal atrophy and weakness with or without sensory symptoms. The resultant atrophy of skeletal muscle is due to the denervation process and could be a potential target for therapeutic intervention with CrM. Traditionally, amyotrophic lateral sclerosis (ALS) has been considered to be in the category of the neuromuscular disorders. ALS is a rapidly progressive neurological disorder affecting predominantly middle- and older-aged adults and leading to alpha motor neuron drop-out with resultant muscle weakness and death in, on average, three years. The topic of neurodegeneration and the potential role for creatine in the treatment of ALS have been well studied in the past and are the subject of chapter 11 (Klein and Ferrante, 2007). A list of some of the more common neuromuscular and neurometabolic disorders is presented in Table 1. The most common peripheral neuropathy in North America and Europe is diabetic predominantly sensory polyneuropathy, with vitamin B12 deficiency being the next most common cause. The main peripheral neuropathy that will be discussed in this chapter will be Charcot-Marie-Tooth (CMT) disease which represents a group of hereditary motor and sensory neuropathies affecting either the myelin sheath or the axons. These patients have progressive distal atrophy with and without clinical sensory loss that initially affects gait but often impairs hand function. The estimated prevalence of CMT disease is 1:2,500 in the population. Table 1. Common neuromuscular disorders . Condition Example A. Myopathy Inherited Muscular dystrophy Duchenne, limb-girdle, myotonic Channelopathy Thomsen’s disease, malignant hyperthermia Acquired Inflammatory Dermatomyositis, inclusion body myositis Toxin Statins, corticosteroids B. Neuropathy Inherited Charcot-Marie-Tooth disease 1A (PMP22 duplication), 1B (MPZ deletion) Spinal muscular atrophy SM1 (smn deletion) Acquired Nutritional deficiency B12, thiamine Diabetes Distal diabetic polyneuropathy Clinical Use of Creatine in Neuromuscular and Neurometabolic Disorders 185 The myopathies represent a heterogenous group of disorders where skeletal muscle is the prime target for dysfunction. In general, the myopathies are classified as hereditary (muscular dystrophy, metabolic myopathies, channelopathies) or acquired (polymyositis, statin-induced myopathy). Most of the research evaluating the potential utility of CrM in neuromuscular disorders has focused on the dystrophinopathies, Duchenne muscular dystrophy (DMD) and Becker’s muscular dystrophy (BMD). DMD is an X-linked progressive myopathy due to mutations within the dystrophin gene, with most boys becoming wheelchair-bound by age 12 y and dying in their early 20s of respiratory or cardiac failure (Brooke et al., 1981a, 1989). The only therapy that has had any beneficial impact on the course of the disease are the corticosteroids (prednisone and deflazacort); however, they are associated with major side effects including osteopenia, dysglycemia, fluid retention, cataracts, and myopathy (Moxley et al., 2005). The neurometabolic disorders represent inborn errors of metabolism affecting intermediary metabolism. For purposes of this chapter, the focus will be on mitochondrial myopathies/cytopathies (e.g., mitochondrial encephalopathy, lactic acidosis, and stroke-like episodes, MELAS), glycolytic and glycogenolytic defects (e.g., McArdle’s disease), and fat oxidation defects (e.g., carnitine palmitoyl transferase deficiency). The mitochondrial cytopathies are a group of disorders with a primary defect of the electron transport chain as the major feature. They can show maternal (MELAS), autosomal recessive (Leigh’s disease) or autosomal dominant (chronic progressive external ophthalmoplegia, CPEO) inher- itance patterns. They occur with a prevalence of about 1:8,000 and reveal extreme clinical and molecular heterogeneity, yet have reduced mitochondrial function/aerobic energy transduction as a common feature. The clinical symptoms include exercise intolerance, muscle weakness, peripheral neuropathy, strokes, migraines, seizures, cardiomyopathy, and ataxia. Defects in glycogenolysis are usually autosomal recessive and include myophosphorylase deficiency (glycogen storage disease [GSD] V, McArdle’s disease) and acid maltase deficiency (GSD II, Pompe’s disease); while an example of a glycolytic defect would be phosphofructokinase deficiency (GSD VII, Tarui’s disease). Patients with these types of muscle GSDs have varying combinations of muscle cramping with exercise, myoglobinuria, proximal weakness (GSD II), and abdominal symptoms (GSD VII). One of the confounding issues when evaluating the neuromuscular and neurometabolic disorders is the fact that these diseases per se lead to inactivity which can lead to disuse atrophy. It is known that disuse of skeletal muscle leads to atrophy, and some of the potential benefits of CrM supplementation could be merely functioning as a counter measure to the atrophy component. Nevertheless, there are a number of fundamental pathologic features that characterize the neuromuscular and neurometabolic disorders that could be amenable to creatine supplementation as outlined below (Table 2). 186 Tarnopolsky Table 2. Potential benefits of creatine monohydrate supple- mentation in neuromuscular and neurometabolic disorders. CHF = chronic heart failure; ↑ = increase; ↓ = decrease . Potential Benefit A. General Weakness ↑ Strength Fatigue ↑ Endurance Muscle atrophy ↑ Muscle mass ↑ Oxidative stress ↓ Oxidative stress B. Neuromuscular-specific ↑ Intracellular Ca2+ ↓ Ca2+ Side effects of corticosteroids ↑ Type II fibers ↓ Mitochondrial function ↑ Mitochondrial function C. Neurometabolic-specific ↑ Risk of strokes CNS anoxia protection Cardiomyopathy Cardiac benefits, ↑ Strength in CHF patients ↓ Mitochondrial function ↑ Mitochondrial function ↓ Phosphocreatine ↑ Phosphocreatine 2. THEORY FOR THE CLINICAL USE OF CREATINE 2.1. Neuromuscular Disorders There are a number of final common pathways that lead to skeletal muscle necrosis, apoptosis and autophagy in myopathies. These final pathways include cytoskeletal disruption, increased reactive oxygen species, electron transport chain dysfunction, increased protein degradation, secondary inflammation and elevated intracellular calcium levels (Mallouk et al., 2000; Pulido et al., 1998; Tarnopolsky and Beal, 2001). A number of studies have demonstrated that CrM supplementation can lead to a reduction in markers of oxidative stress in a variety of tissues, especially the
Details
-
File Typepdf
-
Upload Time-
-
Content LanguagesEnglish
-
Upload UserAnonymous/Not logged-in
-
File Pages22 Page
-
File Size-