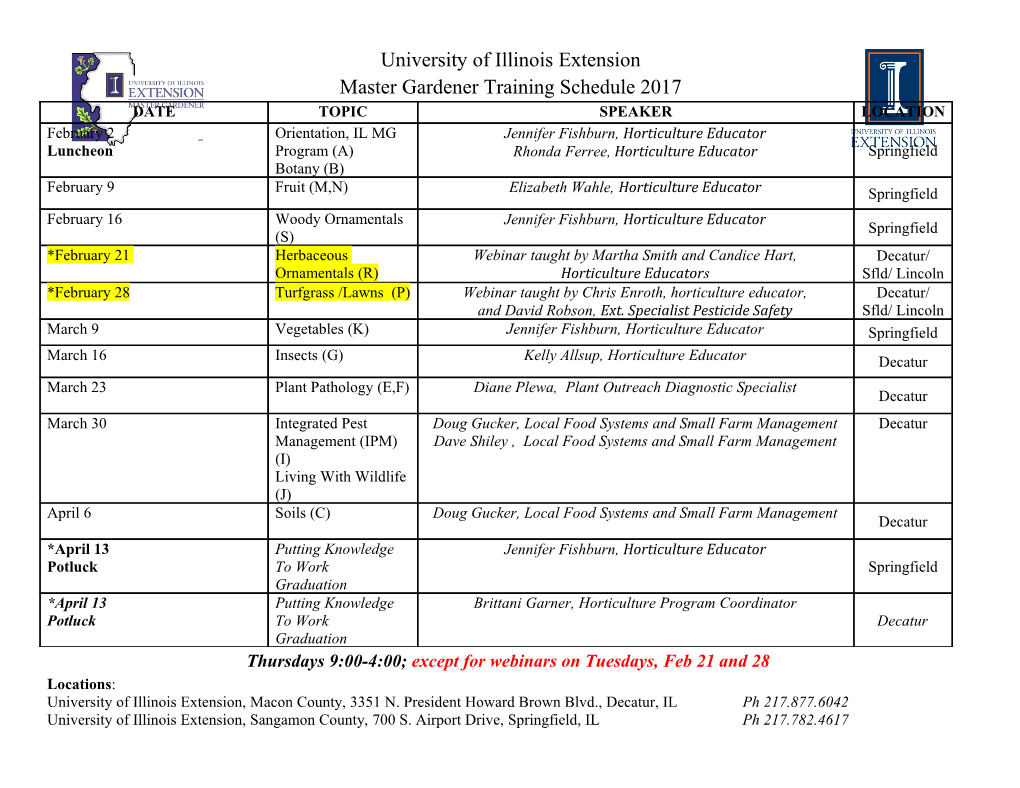
Applied Catalysis A: General 447–448 (2012) 144–150 Contents lists available at SciVerse ScienceDirect Applied Catalysis A: General jo urnal homepage: www.elsevier.com/locate/apcata Hydrodeoxygenation of lignin model compounds over a copper chromite catalyst ∗ Keenan L. Deutsch, Brent H. Shanks Department of Chemical & Biological Engineering, Iowa State University, Ames, IA 50011, USA a r t i c l e i n f o a b s t r a c t Article history: The hydrodeoxygenation of benzyl alcohol, phenol, anisole, o-cresol, catechol, guaiacol, and vanillyl alco- ◦ Received 19 April 2012 hol were carried out from 150 to 275 C at 50 bar H2 with a CuCr2O4·CuO catalyst in a decalin solvent. Received in revised form 5 September 2012 The hydroxymethyl group of benzyl alcohol was found to be highly reactive towards hydrogenolysis Accepted 13 September 2012 to form toluene. Demethoxylation of anisole to form benzene was found to be the primary reaction Available online 8 October 2012 pathway in contrast to demethylation and transalkylation reactions, which are more prevalent for con- ventional hydrotreating catalysts. The hydroxyl group of phenol strongly activated the aromatic ring Keywords: towards hydrogenation forming cyclohexanol which was subsequently dehydrated and hydrogenated Hydrogenolysis Hydrodeoxygenation to form cyclohexane. Reaction networks of increasing complexity were devised for the major functional groups and integrated to describe the most complex molecule studied, vanillyl alcohol. Copper catalyst Lignin © 2012 Elsevier B.V. All rights reserved. Bio-oil upgrading Copper chromite 1. Introduction liquid product from fast pyrolysis [6]. The lignin-derived compo- nents of biomass are commonly used as model compounds for The utilization of biomass to produce fuels and chemicals is a HDO because they possess the aromaticity that is important to topic of increasing importance as petroleum prices rise and reserves maintain to minimize hydrogen consumption [2]. Furthermore, diminish. Numerous technologies are under investigation to utilize phenolic compounds contain several oxygen functionalities includ- the various components of biomass: cellulose, hemicellulose and ing hydroxyl groups bound to aromatic and aliphatic carbons and lignin. A key challenge in the chemical and thermal conversion of methoxy groups that all have different HDO reaction pathways and biomass is the reduction of oxygen content to produce fuels and susceptibilities [7]. chemicals that are compatible with conventional petroleum-based Numerous HDO studies have investigated the use of conven- products. Hydrodeoxygenation (HDO) is a promising upgrading tional hydrotreating catalysts consisting of sulphided NiMo–Al2O3 technology that has gained considerable attention in recent years or CoMo–Al2O3 for bio-oil upgrading [8–32]. Both catalysts have due to the high oxygen content of biomass derived feedstocks, shown to be effective at HDO, where NiMo–Al2O3 favors hydro- especially in the context of pyrolysis oil upgrading [1–5]. Of partic- genated products and CoMo–Al2O3 favors aromatic products. ular importance is the minimization of hydrogen consumption by However, there are several concerns with using sulphided cata- developing catalysts that can perform HDO while minimizing the lysts in HDO such as the loss of the sulphided phase over the hydrogenation of unsaturated phenolic and furanic species that are course of processing and the introduction of sulphur into the prod- common in biorenewable feedstocks. uct stream [11,13,15,21,30,33,34]. Some researchers have proposed Conventional hydrotreating processes have focused primarily co-feeding H2S to maintain the sulphided phase; however, H2S on hydrodesulphurization (HDS) and hydrodenitrogenation (HDN) competitively adsorbs with oxygenated compounds and dispropor- because oxygen content in crude oil is very low and does not tionally decreases the selectivity towards hydrogenolysis, which pose the same environment issues as burning S- or N-containing lowers the production of aromatics. Coking has also been shown fuels [1]. Many studies of HDO have focused on the phenolic com- to be a significant issue with the conventional hydrotreating cat- ponents of bio-oil which represent a considerable portion of the alysts, which has been attributed to the acidity of the support [11,16,17,27,35,36]. Alternatives to the sulphided molybdenum hydrotreating cata- lysts have been investigated in more recent years [33–46]. Noble ∗ metals have shown high activities in HDO; however, aromatic sat- Corresponding author. Tel.: +1 515 294 1895; fax: +1 515 294 1269. E-mail address: [email protected] (B.H. Shanks). uration is observed unless high temperatures and atmospheric 0926-860X/$ – see front matter © 2012 Elsevier B.V. All rights reserved. http://dx.doi.org/10.1016/j.apcata.2012.09.047 K.L. Deutsch, B.H. Shanks / Applied Catalysis A: General 447–448 (2012) 144–150 145 standard of ethanol and identified and quantified using an Agilent GC–MS/FID equipped with a HP-5MS column. The HP-5MS col- umn was unable to separate benzene and cyclohexane; therefore, these two compounds were quantified by m/z values of 78 and 84 respectively. The catalysts were recovered after reaction and dried for 24 h at ◦ 100 C prior to analysis of coking with a Perkin Elmer Simultaneous Thermal Analyzer (STA 6000). This analysis consisted of 5–15 mg of dried catalyst undergoing a catalyst oxidation step followed by a coke oxidation step under 20 mL/min of air flow. A temperature ◦ ◦ ramp from 50 to 250 C at 10 C/min followed by a 120 min dwell was used to completely dry and oxidize the bulk of the copper cata- lysts, which can form passivated oxide layers on the outside of the catalyst upon exposure to an air atmosphere. Following this step ◦ ◦ was a ramp from 250 to 700 C at 5 C/min in which the observed Fig. 1. Primary reactants used for HDO (a) benzyl alcohol, (b) phenol, (c) anisole, (d) o-cresol, (e) catechol, (f) guaiacol, (g) 2-methoxy-4-methylphenol and (h) vanillyl weight loss was attributed to the oxidation of coke. All mass bal- alcohol. ances could be closed within 95% of the total carbon fed to the system. pressures are used to favor aromatic over saturated products. Considering the challenges associated with pyrolysis oil and the 3. Results desired scale of the technologies, a base metal catalyst may be an appropriate alternative to conventional hydrotreating catalysts and 3.1. HDO of mono-functional phenolics noble metal catalysts [5]. Copper mixed metal oxide catalysts are ◦ interesting materials for HDO because they tend to have weak acid- The HDO of phenol was performed at 200–250 C and the ity, high hydrogenolysis activity, and low hydrogenation tendency resulting reaction profiles can be seen in Fig. 2. The reaction for furanic compounds [47–51]. of phenol resulted in the hydrogenation to cyclohexanol as the An important aspect of catalyst research is developing an under- major product. Minor products included benzene, cyclohexene ◦ standing of the reaction network in complex systems such as and cyclohexane. Benzene was not observed at 200 C, whereas the HDO of diverse mono-functional and multi-functional phe- it was observed in a 1:10 and 1:20 ratio to cyclohexane at 225 ◦ nolic species. The current work investigated the HDO of benzyl and 250 C, respectively. Cyclohexene was found at a maxima alcohol, phenol, anisole, o-cresol, catechol, guaiacol, 2-methoxy-4- concentration of less than 1 mM during each run. Cyclohexanol ◦ methylphenol, and vanillyl alcohol with a copper chromite catalyst was also reacted under HDO conditions between 225 and 275 C ◦ from 150 to 275 C at 50 bar H2 in a decalin solvent. The primary and the evolution of cyclohexene and cyclohexane showed the reactants used are shown in Fig. 1. Benzene, toluene, cyclohexanol, same trends as observed in the HDO of phenol. Benzene was ◦ ∼ and methanol were also subjected to HDO conditions to understand detected at a concentration of 0.3 mM by the end of the 275 C the stability of the primary products at high conversions. A reac- run but was not detected at any other sampling point of the reac- tion network for copper was developed which can be contrasted to tions. those of conventional hydrotreating catalysts (S–CoMo–Al2O3 and The product distribution of the HDO of anisole can be seen in S–NiMo–Al2O3) and noble metal catalysts [8,42]. Fig. 3. Anisole was considerably less reactive than phenol and after 20 h of reaction had conversions of 20, 52, and 88 mol% at 225, 250, ◦ and 275 C, respectively. The major products included benzene, 2. Materials and methods cyclohexane, cyclohexanol and methoxycyclohexane. Methanol was not observed by GC. The selectivity towards benzene decreased The following compounds were used in reaction studies or to from 46% to 30 mol% with increasing the reaction temperature from calibrate the mass spectrometer and flame ionization detector of ◦ 225 to 275 C. the gas chromatograph (GC–MS/FID): phenol (Sigma 99%), anisole As seen in Fig. 4, the reactivity of benzene was examined under (Acros 99%), benzyl alcohol (Fisher), guaiacol (Acros 99+%), catechol the same HDO conditions. These data demonstrated that the hydro- (Acros 99+%), o-cresol (Acros 99%), 2-methoxy-4-methylphenol genation of benzene contributed to the loss of selectivity towards (Acros 99%), vanillyl alcohol (Acros 99%), benzene (EMD Chem), benzene under these conditions. Methanol was also subjected to toluene (Fisher ACS), cyclohexane (Fisher ACS), cyclohexanol the same HDO conditions and was observed only in trace amounts (Fisher reagent grade), methanol (Fisher ACS), xylenes (Fisher ACS), at any sampling period. This result was likely caused by the vapor methylcyclohexane (Acros 99%) and decalin (Acros 98%). The cop- pressure exceeding the reaction pressure as methanol approached per chromite catalyst (Acros) was used as received. The catalyst ◦ and became supercritical at 240 C. contained a stoichiometric amount of CuCr2O4 and CuO.
Details
-
File Typepdf
-
Upload Time-
-
Content LanguagesEnglish
-
Upload UserAnonymous/Not logged-in
-
File Pages3 Page
-
File Size-