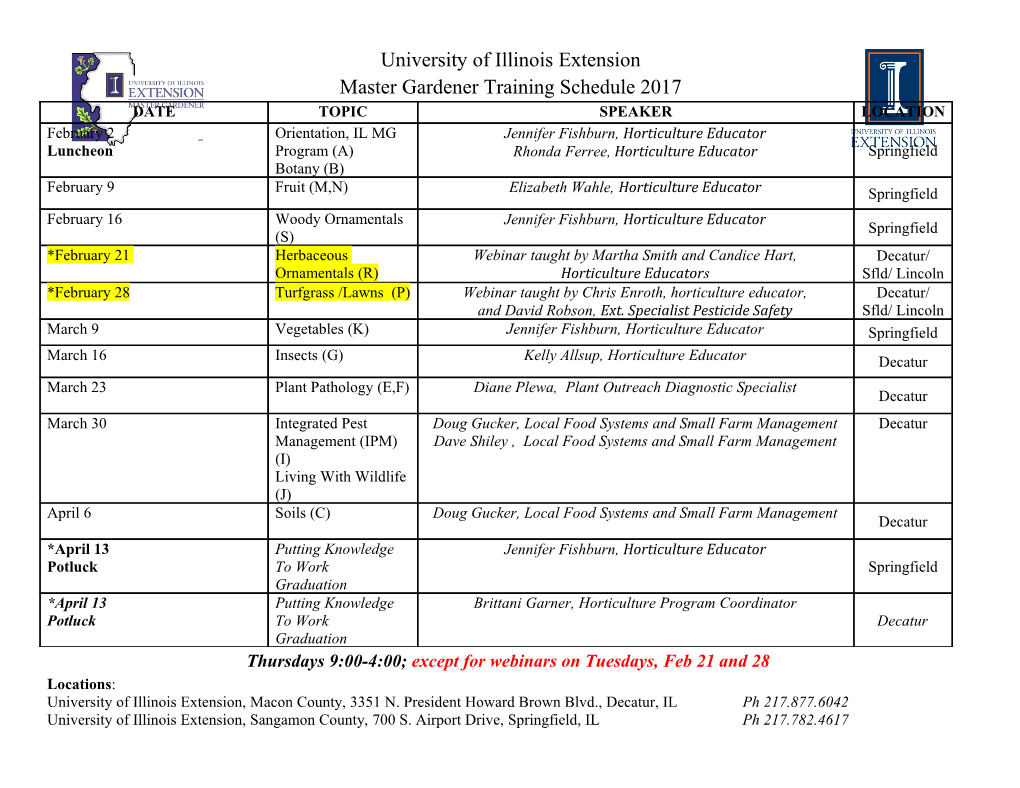
HSJ1 and Motor Neuropathy Heather Louise Smith A Thesis submitted for the degree of Doctor of Philosophy University College London Institute of Ophthalmology 2015 1 Declaration I, Heather Louise Smith, confirm that the work presented in this thesis is my own. Where information has been derived from other sources, I confirm that this has been indicated in the thesis. 2 Acknowledgments I would like to thank my supervisor, Professor Michael Cheetham, for his guidance over the past few years and for allowing me to pursue some of my own research interests. I would also like to thank Professor Alison Hardcastle for her valuable input and advice on the project. A special thanks goes to Naheed Kanuga, for her endless patience and for always having the time to help. I would also like to thank all lab members for their help and assistance and for providing an enjoyable environment to work in. Finally, I would like to thank my family and friends for their encouragement and constant support. 3 Abstract Homo sapiens DnaJ 1 (HSJ1) is a neuronal DnaJ protein that functions to target misfolded and aggregated proteins for degradation. The accumulation of misfolded and aggregated protein can be toxic to cells, causing neurodegeneration; therefore, proteins that function in protein quality control, such as HSJ1, play a critical role in maintaining protein homeostasis and promoting cell survival. The protective nature of HSJ1 is highlighted by the ability of the HSJ1a isoform to combat protein aggregation in cell and mouse models of neurodegenerative diseases, such as amyotrophic lateral sclerosis (ALS), a disease caused by the progressive loss of motor neurons. Based on the anti-aggregation function of HSJ1a towards ALS-associated proteins, I sought to determine whether HSJ1a could also protect against mutant ubiquilin-2 aggregation, a protein recently implicated in X-linked ALS. I identified wild-type ubiquilin-2 as a binding partner of HSJ1a and demonstrated that HSJ1a retained the ability to bind to ubiquilin-2 mutants, suggesting HSJ1a could potentially modulate mutant ubiquilin-2 aggregation in cells. Recently, three mutations in HSJ1 have been identified in autosomal recessive cases of hereditary motor neuropathies, diseases caused by the degeneration of lower motor neurons. I sought to determine the pathogenicity of the most recently identified variant, HSJ1-Y5C. Using a combination of the heterologous expression of HSJ1-Y5C in SK-N-SH neuroblastoma cells and the examination of endogenous HSJ1-Y5C in patient fibroblasts, I characterised the Y5C substitution as a disease-causing, loss of function mutation that reduces the half-life of HSJ1, leading to a loss of protein. To investigate how the loss of HSJ1 function causes motor neuron degeneration, I examined the cellular phenotype of motor neurons in Hsj1 knockout mice. I demonstrated that a significant 12% of motor neurons degenerate between P15 and P20. Prior to motor neuron loss, motor neurons appear chromatolytic and signs of impaired protein homeostasis are observed, with an increase in protein ubiquitylation, a possible increase in autophagosome formation and activation of the IRE1 and PERK branches of the unfolded protein response. The activation of these cellular stress responses suggests that HSJ1 is a critical component of the protein quality control network in motor neurons. 4 Table of contents Title 1 Declaration 2 Acknowledgments 3 Abstract 4 Table of contents 5 List of figures 10 List of tables 12 Abbreviations 13 Chapter 1 – Introduction 1.1 The nervous system 17 1.1.1 Motor neurons 17 1.1.2 The neuromuscular junction 21 1.2 Motor neuron disorders 21 1.2.1 Amyotrophic lateral sclerosis 21 1.2.2 Hereditary spastic paraplegia 21 1.2.3 Spinal muscular atrophy 22 1.3 Inherited peripheral neuropathies 22 1.3.1 Charcot Marie Tooth disease 22 1.3.2 CMT2 and dHMN 23 1.3.3 Mechanisms of disease pathogenesis in CMT2 and dHMN 26 1.3.3.1 Impaired axonal transport 26 1.3.3.2 Mitochondrial dysfunction 27 1.3.3.3 Ion channel dysfunction 28 1.3.3.4 Impaired protein translation 29 1.4 Protein folding 31 1.5 Protein quality control 31 1.5.1 Molecular chaperones 33 1.5.1.1 Hsp90 33 1.5.1.2 Hsp70 34 5 1.5.1.3 DnaJ 37 1.5.1.4 Hsp60 38 1.5.1.5 Small Hsp 38 1.5.2 The ubiquitin proteasome system 39 1.5.3 Autophagy 41 1.5.4 ER-associated degradation 43 1.6 Cell stress responses 43 1.6.1 The unfolded protein response 43 1.6.2 The heat shock response 46 1.7 Impaired protein quality control in neurodegenerative diseases 46 1.7.1 Protein aggregates are a hallmark of neurodegenerative diseases 46 1.7.2 Molecular chaperones are protective in neurodegenerative diseases 47 1.7.3 Mutations in molecular chaperones cause neurodegenerative diseases 49 1.7.3.1 Hsp60 49 1.7.3.2 DnaJC29 50 1.7.3.3 DnaJB2, HSPB1 and HSPB8 50 1.8 Impaired proteostasis in dHMN and CMT2 52 1.8.1 HSPB1 and HSPB8 52 1.8.2 LRSAM1 52 1.8.3 BSCL2 53 1.8.4 DnaJB2 53 1.9 Aims 57 Chapter 2 – Materials and Methods 2.1 In vitro techniques 58 2.1.1 Molecular techniques 58 2.1.1.1 Plasmid transformation 58 2.1.1.2 Plasmid purification (Miniprep) 58 2.1.1.3 Plasmid purification (Midiprep) 58 2.1.1.4 Site directed mutagenesis 59 2.1.1.5 DNA sequencing 62 2.1.2 Cell culture techniques 62 6 2.1.2.1 Reagents 62 2.1.2.2 Cell culture of SK-N-SH cells 62 2.1.2.3 Transfection of 8 well chamberslides 63 2.1.2.4 Transfection of 6 well plates 63 2.1.2.5 Cell culture of human fibroblasts 63 2.1.2.6 The knockdown of HSJ1 in control fibroblasts 64 2.1.2.7 The restoration of HSJ1 in patient fibroblasts 64 2.1.3 Protein based techniques 64 2.1.3.1 Preparation of cell lysates 64 2.1.3.2 Immunoprecipitation 65 2.1.3.3 SDS-PAGE 65 2.1.3.4 Western blot 65 2.1.3.5 Protein quantification using ImageJ 66 2.1.3.6 Proteasome activity assay 66 2.1.3.7 Immunocytochemistry 67 2.1.3.8 Fluorescence microscopy 67 2.1.3.9 Confocal microscopy 67 2.1.4 Quantification of gene expression 67 2.1.4.1 RNA extraction 67 2.1.4.2 cDNA synthesis 68 2.1.4.3 qPCR 68 2.2 In vivo techniques 71 2.2.1 Animal maintenance 71 2.2.2 Genotyping 71 2.2.2.1 GFP PCR 71 2.2.2.2 HSJ1 PCR 72 2.2.3 Transcardial perfusion 72 2.2.4 The preparation of spinal cords for cryosectioning 72 2.2.5 Motor neuron counts 74 2.2.6 Immunohistochemistry 74 7 Chapter 3 – The potential interaction of HSJ1 with UBL proteins 3.1 Introduction 75 3.2 Results 78 3.2.1 HSJ1a co-localises and co-immunopurifies with ubiquilin-2 78 3.2.2 HSJ1a-∆UIM fails to co-localise and co-immunopurify with ubiquilin-2 78 3.2.3 The binding of HSJ1a to ubiquilin-2 is dependent on UIM2 79 3.2.4 Ubiquilin-2 localises to autophagosomes upon the induction of autophagy 83 3.2.5 HSJ1a co-immunopurifies with LC3 under basal conditions and 83 upon the induction of autophagy 3.2.6 Characterising ubiquilin-2 mutants 86 3.2.7 HSJ1a co-localises and co-immunopurifies with ubiquilin-2 mutants 91 3.3 Discussion 95 Chapter 4 – Investigating the pathogenicity of HSJ1-Y5C 4.1 Introduction 101 4.2 Results 105 4.2.1 The Y5C substitution increases the turnover of HSJ1 105 4.2.2 HSJ1-Y5C is targeted to the proteasome for degradation 105 4.2.3 HSJ1-Y5C retains its normal localisation 110 4.2.4 HSJ1a-Y5C retains its anti-aggregation function 110 4.2.5 HSJ1a-Y5C retains the ability to interact with LC3 116 4.3 Discussion 118 Chapter 5 – Investigating HSJ1-Y5C in patient fibroblasts 5.1 Introduction 121 5.2 Results 123 5.2.1 Patient fibroblasts show reduced expression of HSJ1 123 5.2.2 Investigating the effect of the loss of HSJ1 on proteostasis 127 in patient fibroblasts 5.2.3 Optimising the knockdown of HSJ1 in control fibroblasts 130 5.2.4 Optimising the restoration of HSJ1 in patient fibroblasts 130 5.2.5 The lower proteasome activity observed in patient fibroblasts is 135 independent of the loss of HSJ1 5.2.6 The loss of HSJ1 does not activate the UPR in patient fibroblasts 138 8 5.3 Discussion 140 Chapter 6 – Investigating motor neuron degeneration in Hsj1 knockout mice 6.1 Introduction 146 6.2 Results 149 6.2.1 The generation of Hb9:GFP+/- Hsj1+/+ and Hb9:GFP+/- Hsj1-/- mice 149 6.2.2 Characterising GFP expression in Hb9:GFP+/- Hsj1+/+ mice 149 6.2.3 Hb9:GFP+/- Hsj1+/+ and Hb9:GFP+/- Hsj1-/- mice show no 151 difference in the number of motor neurons at P10 and P15 6.2.4 Hsj1-/- mice show a loss of motor neurons between P15 and P20 151 6.2.5 Motor neurons in Hsj1-/- mice show a reduction in area at P15 and P20 152 6.2.6 Hsj1-/- spinal cords show increased levels of ubiquitylated protein 158 6.2.7 The knockout of Hsj1 does not compromise the activity of the proteasome 158 6.2.8 Autophagy is activated in P15 Hsj1-/- mice 161 6.2.9 The presence of chromatolytic motor neurons in P15 Hsj1-/- mice 167 6.2.10 The UPR is activated in Hsj1-/- mice at P10 169 6.3 Discussion 173 Chapter 7 – Discussion 181 References 184 9 List of figures Figure 1.1 The location of upper motor neurons and lower motor neurons 19 in the CNS Figure 1.2 Lower motor neurons project from the spinal cord and directly 20 innervate skeletal muscle fibres Figure 1.3 Examples of CMT2 and dHMN causative genes 30 Figure 1.4 Protein folding free-energy funnel 32 Figure 1.5 Ribbon diagrams showing the structure of representatives from the 35 major molecular chaperone families Figure 1.6 Hsp70 reaction cycle and structure 36 Figure 1.7 Structure of the J domain from E.
Details
-
File Typepdf
-
Upload Time-
-
Content LanguagesEnglish
-
Upload UserAnonymous/Not logged-in
-
File Pages205 Page
-
File Size-