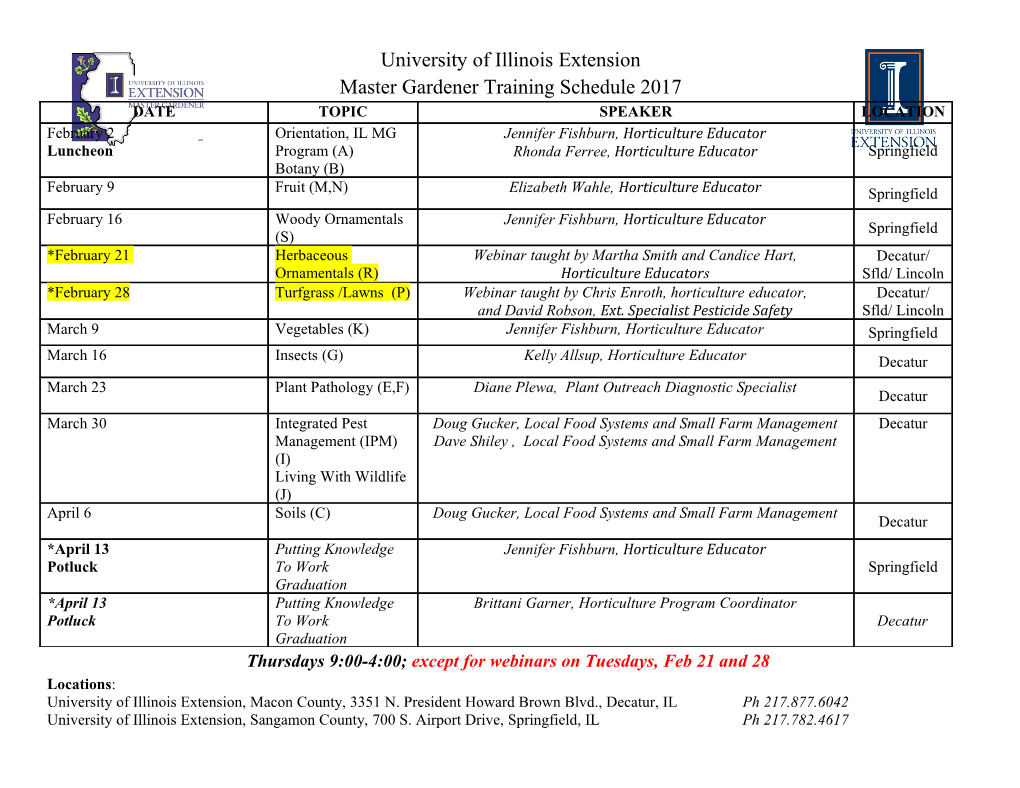
Space science applications of cryogenic detectors N.Rando, D.Lumb, M.Bavdaz, D.Martin, T.Peacock Science Payload & Advanced Concepts Office, European Space Agency, ESTEC, Noordwijk, The Netherlands Abstract The improved performance of cryogenic detectors has drastically enhanced their utilization range, allowing a number of space-based applications, with particular emphasis on astronomical observations. In this paper we provide an overview of the main applications of cryogenic detectors onboard spacecraft, together with a description of the key technologies and detection techniques used or being considered for space science missions. A summary of the cryogenic instrumentation technologies is also presented. Specific emphasis is given to space based astronomy in the soft X- ray regime, where superconducting tunnel junctions and cryogenic calorimeters offer well identified advantages. Possible instruments for future astrophysics space missions are also discussed, using XEUS (X-ray Evolving Universe Spectroscopy mission, presently proposed by ESA as a post XMM-Newton project) as a reference. To be published in the proceedings of the 2nd workshop on advanced Transition Radiation Detectors for accelerator and space applications (TRD 2003), held in Bari (Italy) on September 4- 7, 2003. Introduction Cryogenic detectors and cooling technologies have made a remarkable amount of progress over the last 20 years. The increased reliability and simplicity of operations of cryogenic equipment have allowed to install and operate them onboard spacecrafts, while the improved performance of cryogenic devices, such as sensors and cold electronics, has opened new applications [1]. Remarkable progress has been achieved in the fabrication of thin film based micro- devices (Low Temperature Superconductors such as Nb, NbN, Ta) showing highly uniform properties and excellent resistance to thermal cycles. Such devices include Superconducting Tunnel Junctions (STJs), SQUIDS (Superconducting Quantum Interference Devices) and simpler components, such as resonating cavities and low losses RF filters. Albeit at a lower pace than initially predicted, also High Temperature Superconductors are moving from a pioneering phase to more mature technological applications. In particular, the advent of radio-frequency devices based on HTS has opened up new perspectives for space-borne applications, in view of more energy efficient and better performing telecommunication platforms. Over the last 15 years several space missions involved cryogenic equipment, mostly in relation with astrophysics missions, at a wavelength range otherwise difficult to work with from ground. Among such missions we should mention Einstein (NASA’s X-ray observatory launched in 1978) [2], IRAS (Infrared Astronomical Satellite, launched in 1983), COBE (Cosmic Background Explorer, launched in 1989) and ISO (Infrared Space Observatory, launched in 1995) [3]. The Japanese satellite Astro-E (X-ray observatory) was designed to carry a spectrometer (XRS) built by NASA and operating at a temperature of 65 mK [4]. At this moment, several space missions involving cryogenic applications are in advanced development, in Europe as well as in Japan and US. Good examples are the ESA space science missions Planck (dedicated to the mapping of the cosmic background radiation) and Herschel (formerly known as FIRST- Far Infrared and Submillimetre Telescope) [5]. Both satellites will carry scientific payloads working at temperatures of 0.1 K and 0.3 K respectively. SIRTF (Space Infrared Telescope Facility, launch in August 2003), one of the four large NASA’s observatories, is based on a 4He cryostat and it will perform photometry, spectroscopy and imaging in the 3 – 180 µm spectral range [6]. On longer time-scales (post 2010-2015), other space missions with cryogenic equipment are under study, usually targeting low operating temperatures (down to 50- 100 mK) and long lifetimes (5-10 years), such as ESA’s XEUS [7] (a post XMM mission) and NASA’s Constellation-X (the High Throughput X-Ray Spectroscopy mission), both envisaging micro-calorimeters working at T<100 mK. In the higher temperature range, between 100 and 10 K, many missions are already operational or in development. They include military reconnaissance satellites (such as Helios), earth observation satellites (SPOT) and meteorological spacecrafts (MSG, Meteosat Second Generation), with IR detectors operating at about 85 K. Cryogenics in Space This section provides a brief review of the main scientific missions using cryogenics in chronological order, starting from Einstein and IRAS, the first ‘cryogenic missions’, which flew respectively in 1978 and 1983. Mission in operations (or post- operations), missions presently under development and missions under study are grouped in different sections of table 2 provides a summary of all space missions that involve cryogenics. Additional details can be found in [1]. - Missions in operations / post-operations. Einstein (HEAO-2) was the second of NASA’s three High Energy Astrophysical Observatories and the first X-ray telescope put into space in 1978. Among other instrument, Einstein carried a Solid State Spectrometer using a Si(Li) crystal detector (range 0.5-4.5 keV) cooled at about 100K via a solid ammonia/methane cryostat [2]. IRAS (Infrared Astronomy Satellite, launched in 1983) was the first cryogenic scientific satellite. Its mission was to map the entire sky from 8 to 120 µm and it was equipped with a 0.6 m telescope cooled with liquid He to about 4 K. The focal plane assembly operated 62 photo-conductive elements at 3K. COBE (Cosmic Background Explorer) was developed by NASA’s to measure the cosmic background radiation. The satellite was launched in November 1989. It carried three instruments, operating at wavelengths between 1.25 and 240 µm with focal planes at 1.6 K, cooled by a 650 litre superfluid helium cryostat. ESA’s ISO (Infrared Space Observatory) operated at wavelengths from 2.5 to 240 µm between November 1995 and May 1998 in a highly elliptical orbit. The satellite is based on a cryostat containing about 2200 litre of superfluid helium and on a 0.6 m diameter telescope. The instruments made use of different photo-conductors based on InSb, Si and Ge and operating between 1.8 and 10 K [3]. NICMOS (Near Infrared Camera and Multi-Object Spectrometer) is a Hubble Space Telescope (HST) instrument based on three cameras designed for simultaneous operations, operating between 0.8 and 2.5 µm and using HgCdTe photo-conductive detectors, cooled down to 50-60 K via 120 kg of solid nitrogen. A mission study involving cryogenic equipment (not selected for flight) is represented by the ESA’s STEP (Satellite Test of the Equivalence Principle), aiming to verify the equivalence of gravitational and inertial mass to a precision of one part in 1017. Such a precision would have been guaranteed by superconducting accelerometers cooled at 1.8 K by a bath of superfluid He. WIRE (Wide Field Infrared Explorer), one of the NASA small explorers, was launched in February 1999 and lost during its commissioning phase. It was supposed to survey the sky at mid-infrared wavelengths, between 12 and 25 µm. A two-stage, solid hydrogen cryostat maintained the optics colder than 19 K and the 128x128 Si:Ga detector array below 7.5 K. Astro-E (NASA and Japan’s effort) is a satellite for X-ray astronomy launched in 2000 and not operational due to a launcher problem. Astro-E was designed to provide X-ray images along with high resolution spectra from 0.4 to 700 keV. One of the instruments onboard, was based on an array of 2x6 micro-calorimeters, operating at 65 mK. Such a temperature is maintained by an ADR (Adiabatic Demagnetisation Refrigerator) hosted in a liquid helium cryostat and thermally shielded by solid-neon cooled outer jacket [6]. INTEGRAL (International Gamma-Ray Astrophysics Laboratory, a medium size ESA science mission dedicated to spectroscopy and imaging between 15 keV and 10 MeV) was launched in December 2002 and uses space qualified Stirling cryo-coolers [8]. The spectrometer installed on the spacecraft (SPI) is based on about 30 kg of germanium detectors maintained at a temperature of 85K. The satellite is presently operating nominally with an expected lifetime extending to 2005. - Missions under development. ESA is presently developing (phase C/D, 2003) two important cryogenic astronomical missions: Planck and Herschel [5]. Planck’s main objective is to map the temperature anisotropies of the Cosmic Microwave Background (CMB) over the whole sky, with a sensitivity ∆T/T = 2.10-6 and an angular resolution of 10 arc-minutes. Such goals require bolometers operating at 0.1K, HEMT at 20 K and a cooled telescope (60 K). Planck’s cryogenic system uses pre-cooling to 60 K by passive radiators, cooling to 20 K with a H2 Joule Thomson Cooler (adsorption compressors), cooling to 4 K with a He Joule-Thomson cooler (mechanical compressors) and final cooling to 0.1 K with an open loop Dilution Refrigerator. Mission lifetime is 15 months. Herschel (formerly known as FIRST, see figure 1) is dedicated to astronomical observations in the far-infrared and sub-mm wavelength range, from 85 to 600 µm. Herschel is based on a superfluid helium dewar at 1.65 K and on a 3He sorption coolers delivering a base temperature of 0.3 K. The scientific goals will be achieved with three instruments operating respectively at 2 K (HIFI, heterodyne receiver based on SIS mixers), 1.7 K (PACS, spectro-photometer partly based on photo-conductors) and 0.3 K (again PACS and SPIRE, another spectro-photometer using bolometers). Herschel is presently scheduled for launch in 2007 with a mission lifetime of 4.5 year. NASA’s SIRTF (Space Infrared Telescope Facility) is designed to perform imaging and spectroscopy in a large wavelength range, from 3 (NIR) to 180 (FIR) µm via a 0.85 m diameter, helium cooled telescope [6]. The detectors’ temperature is 1.4 K, while the cryogenic system makes use of 360 litre of superfluid He, for a lifetime of 2.5 years.
Details
-
File Typepdf
-
Upload Time-
-
Content LanguagesEnglish
-
Upload UserAnonymous/Not logged-in
-
File Pages17 Page
-
File Size-