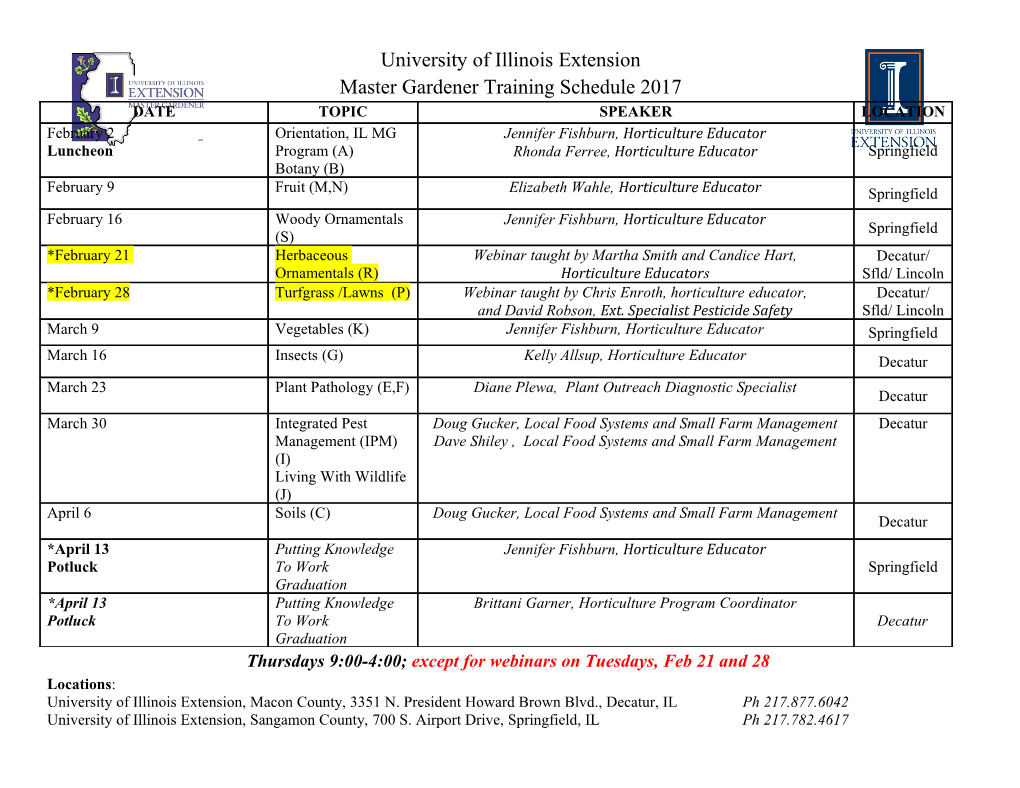
Universität zu Lübeck SS 2012 Interdisziplinäre Vorlesung zur Lasermedizin Prof. Alfred Vogel Laserdisruption: Plasma-mediated surgery Outline: Plasma-mediated surgery . Principle and applications of intraocular surgery 1 . Plasma-mediated fragmentation: laser lithotripsy . Femtosecond laser cell surgery 2 . Nanocavitation for cell transfection 3 . Plasma-mediated transport of biomaterials Mechanical damage and bleeding from explosive vaporization induced by linear absorption at the RPE Light absorption Localized energy of cells & tissues deposition Absorption coefficients of biological tisues DIC image of cells DIC images of cells in culture medium Localized energy deposition within transparent tissues or cells requires: - wavelengths that can penetrate, Laser effects - tight focusing, and in cornea - nonlinear absorption Example: Intraocular dissection and disruption Iridotomy Capsulotomy after cataract surgery Conflicting tasks: Light must penetrate into the eye + localized energy deposition nonlinear absorption required ! Discovery of “laser lightning” 1963 Maker et al. observed plasma sparks when „giant“ ruby laser pulses were focused in air 12 2 Threshold 10 W/cm Name: „optical breakdown“ 1964 Discovery of optical breakdown in liquids and solids Transmission Optical breakdown = nonlinear absorption through laser focus I Local energy deposition within transparent dielectrics becomes possible (Meyerand & Haught, Phys. Rev. Lett. 1964) Laser-lightning Energy deposition by DC breakdown nonlinear absorption 11 2 3 2 (I 10 W/cm coagulation: 10 W/cm ) Laser pulse Large irradiance in laser focus leads to localized energy deposition via plasma formation („optical breakdown“) The pulse duration must be short (≤ ns) to minimize the amount of deposited energy Energy density in luminescent ns plasma: 40 kJ/cm3 Vaporization enthalpy of water: 2.6 kJ/cm3 Energy density of TNT: 6.9 kJ/cm3 Devices and considerations for laser treatment Plasma-mediated intraocular laser surgery - ‘no hole’ surgery - Iridotomy Capsulotomy Dissection of a vitreal strand to stop retinal traction Dynamics of plasma formation (“Optical breakdown”) E Inverse bremsstrahlung absorption Kinetic E energy Avalanche Quasi-free state Band gap E Bound state Photo- Impact t ionization ionization • Initial free electrons are generated by photoionization (multiphoton ionization or tunneling) • Free electrons gain energy by „inverse bremsstrahlung absorption“ of photons • When the free electrons have gained sufficient kinetic energy, they produce more free electrons by impact ionization ionization avalanche Wavelength dependence of threshold portrays interplay of multiphoton and avalanche ionization 5,0 ) ² 4,5 Average values for NA = 0.8 and NA = 0.9 4,0 and 2 ns pulse duration W/cm 11 3,5 4 photons 3,0 5 photons 2,5 6 photons 2,0 1,5 1,0 band gap: 6.6 eV 0,5 Irradiance threshold (10 0,0 650 700 750 800 850 900 950 1000 1050 1100 Wavelength (nm) Breakdown threshold increases with number of photons required for MPI Rate of avalanche ionization increases with 2 Plasma grows above threshold by ‘breakdown wave’ Power 3 2 Pth 1 Time Laser 1 2 3 light Laser pulse 20 µm T > 10 000 K Shock wave emission 6 ns , 1064 nm, 10 mJ 100 µm Shock wave velocity and pressure 6 ns, 5 mJ plasma 100 µm 10 ns (us c0 )c2 Shock wave velocity shock wave pressure: ps c1 0 us 10 1 p Shock velocity Pressure Sound velocity : Initial pressure at plasma rim: 100 kbar Cavitation bubble dynamics 90 ns 300 µs 1 mm 130 µs Expansion velocity > 500 m/s for 30-50 ns Jet velocity: 100 m/s IR ns breakdown is highly disruptive Ns laser pulse focused on 6 ns, 10 mJ corneal endothelium and Descemet’s membrane R S 0.8 % 0.5 % 6 mJ Ein T 100 % 2.6 % A 96.1 % ES EB EV ER ? 58.9 % 29.4 % 6.5 % 6 x 10 -4 % 1.3 % Mechanical energy Vaporization energy 88.3 % 6.5 % 100 µm Tissue effects by shock waves Short duration of Elasticity of tissue shock wave (<20ns) Tissue translations < 5 µm, no gross tissue effects (They are caused by the cavitation bubbles) but possibly damage to cell organelles and membrane and Lose to plasma phase transitions at shock front Tissue effects by plasma and bubbles Disintegration Jet of tissue within Impact plasma volume Strategies to minimize side effects Correct placement of plasma center Small pulse energies to minimize collateral damage Capsulotomy Dissection of vitreoretinal strands Parameter optimization (1) TEM multimode Multiple plasmas laser beam TEM (0/0) single mode laser beam Well localized single plasma Parameter optimization (2) Shorter pulses higher precision Reduction of pulse duration from ns to fs : Lower energy threshold 6 ns for optical breakdown Finer tissue effects 1000 1000 F I ) th ) Ithth Fth -2 -2 30 ps 100 µm Graph 100 100 W cm 10 10 10 Irradiance (10 Radiant exposure (J cm 1 1 100 fs 10 ps 1 ns 100 ns PulsePulse duration duration Iridotomies produced using different laser pulse durations 6 ns, single exposure 40 ps, many pulses Flap cutting for corneal refractive surgery Correction of myopia... Traditional Flap generation for LASIK ... by ArF excimer laser ablation Fs Flap generation (large NA) Femtosecond laser 100 fs 1 mm Microkeratome Methods of refractive femtosecond laser surgery Fs Flap generation for ArF-laser LASIK Lenticule cutting and removal Fs-keratome: Reproducible flap thickness Freedom in cutting geometry flap can be designed for precise re-positioning after ArF laser surgery ! important for wavefront-guided surgery ! History of LASIK (Video) Bis hierher klausurrelevant. Das Folgende ist Zusatzmaterial Principal requirements for controlling the laser lightning . Small aberration-free laser focus . Precise and smooth tunability of deposited energy . Good pulse-to-pulse reproducability In which part of the parameter space of pulse duration and laser wavelength can these requirements be fulfilled? Have another careful look (after 40 years of research on optical breakdown), both on an experimental and a theoretical level ! Experimental requirements 0,40 0,35 0,30 Laser 0,25 0,20 • Excellent beam quality (temporal and spatial) 0,15 0,10 0,05 0,00 Setup -1,0 -0,5 0,0 0,5 1,0 1,5 • Tight focussing (large NA), to achieve a small focus suitable for the creation of nanoeffects • Aberration-free focussing in spite of large NA (UV-VIS-IR water immersion-objectives) • Water as breakdown medium (less impurities than in solids) Breakdown criterion • Bubble formation instead of plasma luminenscence (sensitive, well defined, easily detectable) Pulse shapes of Nd:YAG ns-laser pulses - regular versus Gaussian (slm) pulses - 0,10 unseeded 1064 nm unseeded 532 nm 0,15 seeded t = 11.2 ns seeded t = 8.8 ns L 0,08 L 0,10 0,06 0,04 0,05 Amplitude (V) Amplitude (V) 0,02 0,00 0,00 -2,00E-008 0,00E+000 2,00E-008 -2,00E-008 0,00E+000 2,00E-008 Time (s) Time (s) unseeded 355 nm 0,20 seeded tL = 6.7 ns Smooth pulse shape good reproducability 0,15 0,10 Plasma generation by smooth Amplitude (V) 0,05 ns pulses is “deterministic”, similar to femtosecond breakdown! 0,00 -1,00E-008 0,00E+000 1,00E-008 Time (s) Bubble detection & determination of bubble size • Theory predicts (at threshold) flash lamp (18 ns) bubble diameter < 200 nm (Appl Phys B 81:1015-1047, 2005) Bubble detection via laser pulse light scattering photo- (Phys. Rev.Lett. 100:038102, 2008) detector cw probe laser E = 19,5 nJ 20 = 90 ns NA = 0.5 Light deflection by bubble • Determination of bubble radius R by measuring the bubble max CCD camera E = 21,7 nJ oscillation time T E = 24 nJ E = 42 nJ = 300 ns • Minimum detectable radius photographs: 850 nm T p0 pv Rmax Scattering technique: < 50 nm 1.83 0 E = 19,8 nJ = 130 ns Bubble size for fs pulses and regular ns pulses (40x, NA = 0.8) 1000 300 fs 7-11 ns 1064 nm Regime for capsulotomies 532 nm & iridotomies 100 355 nm 1040 nm 532 nm 10 1,0 346 nm 0,8 0,6 1 0,4 amplitude [a.u.] Bubble radius (µm) 0,2 = 1064 nm 0,0 FWHM 11 ns -30 -20 -10 0 10 20 30 0,1 time scale [ns] 1E-3 0,01 0,1 1 10 100 1000 Pulse energy (µJ) Fine femtosecond-effects Gross nanosecond-effects “deterministic” “stochastic” Stepwise breakdown with smooth UV ns pulses 355 nm, 6.8 ns, 40x, NA = 0.8 Bubble- and plasma size 1000 Bubble 100 diameter 10 Size (µm) 1 Plasma length 0,1 0 20 40 60 80 100 Pulse energy (µJ) 20 µm Energy and pulse duration dependence of bubble size (40x, NA = 0.8, Gaussian ns pulses) 1000 7-11 ns 0.5 – 1.0 ns 1064 nm 100 300 fs 1064 nm 1040 nm 520 nm 10 346 nm 355 nm 355 nm 532 nm 1 Bubble radius (µm) 532 nm 0,1 1E-3 0,01 0,1 1 10 100 1000 Pulse energy (µJ) Microchip lasers can do what could previously be achieved only by using expensive femtosecond lasers ! 100 350 fs 10 0.5 - 0.9 ns 350 fs, 1040 nm, NA 0.9 1 350 fs, 1040 nm, NA 0.8 0.5 ns, 355 nm, NA 0.9 Bubble radius (µm) 0.5 ns, 355 nm, NA 0.8 0.9 ns, 355 nm, NA 0.9 0.9 ns, 355 nm, NA 0.9 0,1 1 10 Normalized pulse energy E / Eth The small-bubble regime is larger with ns pulses than with fs pulses Good tunability of laser effects Can we utilize the new level of understanding of nonlinear energy deposition for improving medical technology ? Flap creation in refractive surgery (LASIK) Mechanical keratome Femtosecond laser • one cutting pattern • various cutting patterns • precision & complication rate • high precision & reproducability depends on surgeon’s skills • few complications • small • bulky • cost-effective • expensive New approach for flap creation 5 mm flap, porcine eye • UV-laser (355 nm, 600 ps) 200 µm • 150 kHz pulse repetition rate • good beam quality • compact Development of a commercial
Details
-
File Typepdf
-
Upload Time-
-
Content LanguagesEnglish
-
Upload UserAnonymous/Not logged-in
-
File Pages90 Page
-
File Size-