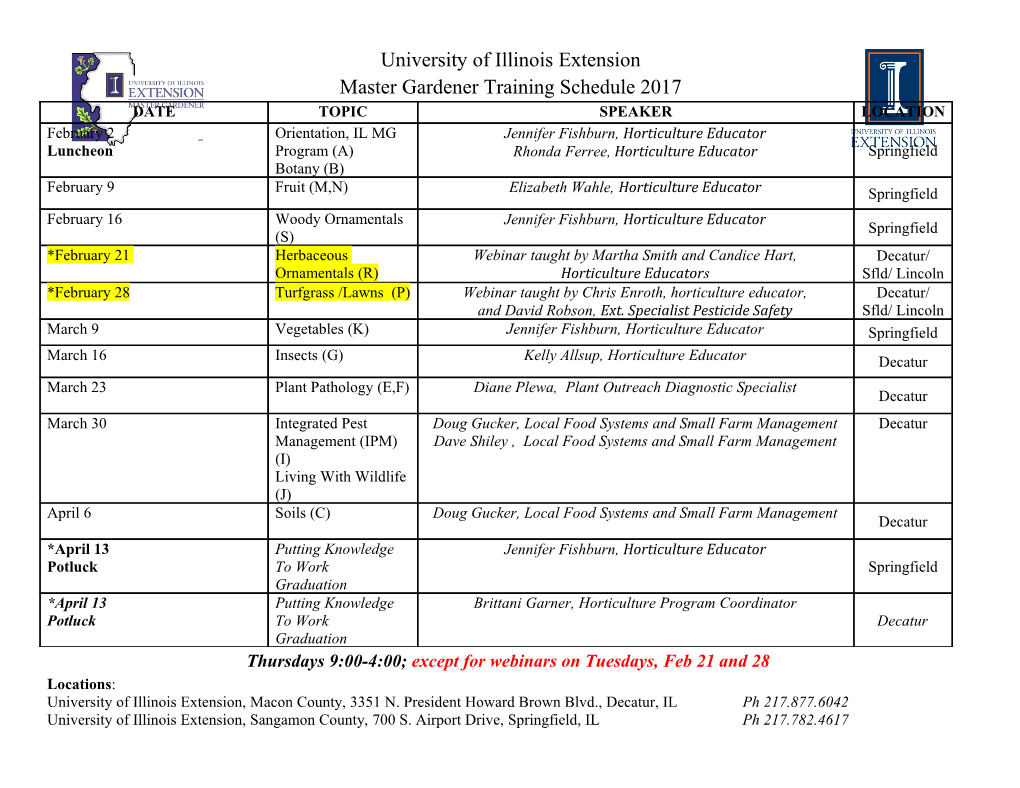
Improved performance of metal hydride electrode of Ni-MH battery with carbon nanotubes by Humara Sultana Submitted to the School of Materials Science and Engineering in partial fulfillment of the requirements for the degree of Master of Science at The University of New South Wales, Australia 2006 Abstract Abstract In the global search for renewable sources of energy, hydrogen is a promising candidate in transportation and electronic applications. Carbon nanotubes (CNTs) have the largest hydrogen storage capacity among the hydrogen storage materials known at present. The Ni- MH battery can be used to store and then discharge large amounts of hydrogen reversibly by using hydrogen storage materials as negative electrode. The electrochemical hydrogen storage performances of metal hydride electrodes with different levels of multi wall carbon nanotubes (20%, 15%, 10%, 5% and 2% of Ni-MH battery’s active materials) has been investigated under similar charge-discharge conditions. Electrochemical test cell consisted of a single hydrogen storage negative electrode sandwiched between two NiOOH/Ni(OH)2 positive electrodes. A 6M aqueous KOH solution was used as electrolyte. Electrochemical properties such as specific discharge capacity, high rate charge-discharge capability and cycle life stability have been investigated. The morphology and structure of negative electrode material were examined by scanning electron microscopy, transmission electron microscopy and X-ray diffraction analysis. Chemical analysis of the hydrogen storage alloy was performed using electron probe microanalysis, electron diffraction spectroscopy and induced coupled plasma spectroscopy analysis. Hydrogen absorption-desorption properties were measured in terms of pressure-composition-isotherm curves. It has been found in this study that the presences of CNTs significantly enhanced the overall electrochemical properties of the Ni-MH battery. Maximum specific discharge capacity was observed for 5% CNTs electrode reaching 243 mAh/g, whereas 0% CNTs could only reach 229 mAh/g. High rate charge and discharge capabilities of 5% CNTs I Abstract electrodes were ~ 241% and 250% higher than the corresponding values for 0% CNTs electrode. Furthermore, the differences in electrochemical hydrogen storage of CNTs with different diameters of 10-20 nm, 20-40 nm, 40-60 nm, and 60-100 nm were investigated. Electrochemical results demonstrated that CNTs with different diameters showed a large variation in the electrochemical hydrogen storage capability under the similar experimental condition. A comparison between electrodes with different CNTs studies was carried out in order to optimize nanotubes choices for Ni-MH battery. It was found that smaller tube diameters, 20-40 nm and 5% CNTs negative electrode showed the best electrochemical properties of Ni-MH battery system. II Acknowledgements This thesis is the result of the inspiring, thoughtful and patient guidance of my supervisor Senior lecturer Dr. Sammy Lap Ip Chan over the last two years. My sincere gratitude goes to my mentor and friend Dr. Rita Khanna for her valuable suggestions, helpful research support, ideas, and inspirations. Without her kind and sincere help this thesis would not be possible. My special thanks go to Professor Oleg Ostrovski, head of the School of Materials Science and Engineering, UNSW, for his guidance and support towards the completion of this work. I am ever grateful to my friends: Tawfique Hasan, Susan Lee, Jerry Luo, Leida Queddeng, Farjana Sarwar, Mrs Emon Rahman, MD. Faruqe for making my life in the university and in Sydney a vibrant and truly enjoyable one. Friendly and hard-working faces of fellow postgraduate students Muhammed Mahfuzur Rahman, Mahburur Rahman, Gavin Parry surely kept me awake during numerous sleepy afternoons. I am very grateful to the University of New South Wales for providing me with scholarship and opportunity to doing this research. I would like to extend my appreciation to the Professors, staffs and students of School of Materials Science and Engineering for their support and encouragement. And finally, I would like to thank my parents and in laws, brothers, sisters and my beloved husband Faisal Sarwar for everyday sacrifices and support in this adventure. Humara Sultana iii Dedication To my parents and in laws. iv Table of contents Chapter 1: Introduction 1 1.1 Background 1 1.2 Problem facing Ni-MH batteries 2 1.3 Project objectives 3 1.4 Thesis overview 5 References 7 Chapter 2: Literature review 9 2.1 Introduction 9 2.2 Hydrogen as a clean energy source 9 2.2.1 What is carbon nanotube? 10 2.2.2 CNTs as a mean of hydrogen storage 12 2.2.3 Metal hydrides as a mean of hydrogen storage 15 2.3 Ni-MH battery 18 2.3.1 Comparison of rechargeable battery system 19 2.3.2 Reaction mechanism of Ni-MH battery 21 2.4 Electrochemical performance of Ni-MH battery 23 2.4.1 Discharge capacity of negative electrode 24 2.4.2 Activation profile and cycle life test 26 2.4.3 Self-discharge 31 2.4.4 High rate charge-discharge capabilities 32 2.5 PCI measurements 35 References 37 V Chapter 3: Experimental details 43 3.1 Introduction 43 3.2 Sample details 43 3.2.1 Materials 43 3.2.2 Experimental electrodes 46 3.3 Sample characterization techniques 47 3.4. Preparation of electrodes 49 3.5 Current collector 53 3.6 Electrochemical test cell setup 52 3.7 Electrolyte soaking of electrodes 54 3.8 Apparatus for electrochemical measurements 54 3.9 Electrochemical testing parameters for negative electrode 55 3.10 Experimental procedure 56 References 65 Chapter 4: Results and discussion 67 4.1 Introduction 67 4.2 Surface morphology 67 4.2.1 SEM, EDS and EPMA analysis 67 4.2.2 TEM analysis 74 4.3 Structure analysis 75 4.3.1 Crystal structure analysis 75 4.3.2 Structure analysis of CNTs 79 4.4 Electrochemical measurements 80 4.4.1 Activation and maximum capacity 81 VI 4.4.2 Discharge potential analysis 86 4.4.3 Cycle life characteristics and cycle stability 88 4.4.4 SOC, DOD and self-discharge test 98 4.4.5 High rate discharge characteristics 103 4.4.6 High rate charge characteristics 108 4.5 PCI measurements 111 4.6 Limitations of this study 113 References 115 Chapter 5: Conclusions 121 5.1 Conclusions 121 5.2 A brief summary of main results 121 5.3. Future work directions 125 5.4 Potential applications in electric vehicles 127 References 128 Appendix: Electron probe micro analysis spectrum 129 VII List of Figures Fig. No. Figure caption Page Fig. 2.1 Schematic diagram of carbon nanotubes. 11 Fig. 2.2 The power density versus energy density plot distinguishes various 14 electricity storage systems. Fig. 2.3 Schematic diagram of the electrochemical reaction process of a Ni- 22 MH battery. Fig. 2.4 The discharge curves of LaNi5 electrodes doped with different ratios 25 of CNTs of 15%, 10%, 7% and 5% in cycle number 40. Fig. 2.5 Variation of discharge capacity of the two samples with number of 25 charge-discharge cycles at 25° C. Fig. 2.6 Activation profile of La0.85MgxNi4.5Co0.35Al0.15 hydride electrodes. 27 Fig. 2.7 The hydrogen storage curves of CNTs-Ni electrode with different 27 diameter. Fig. 2.8 Discharge curve of CNTs-LaNi5 electrode of 20-40 MWNTs treated 30 at different temperatures in nitrogen. Fig. 2.9 Discharge curve of CNTs-LaNi5 electrode with different diameter 30 CNTs treated with 500° C in Vacuum. Fig. 2.10 Storage characteristics of Ni-MH battery at different temperature. 31 Fig. 2.11 A solar vehicle used for Ni-MH study, Japan. 33 Fig. 2.12 Charge efficiency of the MH alloy sample. 34 Fig. 2.13 High rate discharge capability of the different electrodes. 35 VIII Fig. 3.1 Schematic diagram of experimental negative electrode. 49 Fig. 3.2 Photograph of the prepared negative electrode. 49 Fig. 3.3 Flow chart of the preparation of pasted type negative electrode. 50 Fig. 3.4 Photograph of as received positive electrode. 51 Fig. 3.5 The schematic diagram of the improved original positive electrode. 51 Fig. 3.6 Schematic diagram of cell assembly. 53 Fig. 3.7 Photograph of charge-discharge REPOWER machine. 54 Fig. 3.8 Flow chart of the experimental test of the negative electrodes. 56 Fig. 3.9 Experimental procedure of the electrochemical measurements. 56 Fig. 3.10 Sketch map of PCI system suitable for hydrogen storage materials 63 used in this experiment. Fig. 4.1 SEM analysis of 0% CNTs electrode (upper picture) and 5% CNTs 68 electrode (lower picture). Fig. 4.2 SEM image of ground 10% CNTs, Ni and AB5 alloy. 69 Fig. 4.3 The SEM image of as received AB5 alloy. 69 Fig. 4.4 EDS analysis of as received AB5 alloy. 70 Fig. 4.5 SEM image of as received CNTs diameter ranging from 20-40 nm. 73 Fig. 4.6 SEM image of as received polypropylene separator. 73 Fig. 4.7 TEM image of as received CNTs, diameter ranging from 20-40 nm. 75 Fig. 4.8 Schematic diagram of the crystal cell of AB5 alloy. 76 Fig. 4.9 XRD pattern of as-received AB5 alloy. 76 Fig. 4.10 XRD diffraction of as received CNTs, diameter ranging from 20-40 77 IX nm and ball milled CNTs (1hour) (upper graph) and 10-20 and 60- 100 nm CNTs (lower graph). Fig. 4.11 XRD diffraction of as received Ni powder. 78 Fig. 4.12 XRD diffraction of prepared CNTs electrodes. 79 Fig. 4.13 Raman spectrum of as received CNTs, diameter ranging from 20-40 80 nm. Fig. 4.14 Activation profile of different kind of CNTs electrode. 81 Fig. 4.15 Effect of diameter and ball milled CNTs on activation profile.
Details
-
File Typepdf
-
Upload Time-
-
Content LanguagesEnglish
-
Upload UserAnonymous/Not logged-in
-
File Pages151 Page
-
File Size-