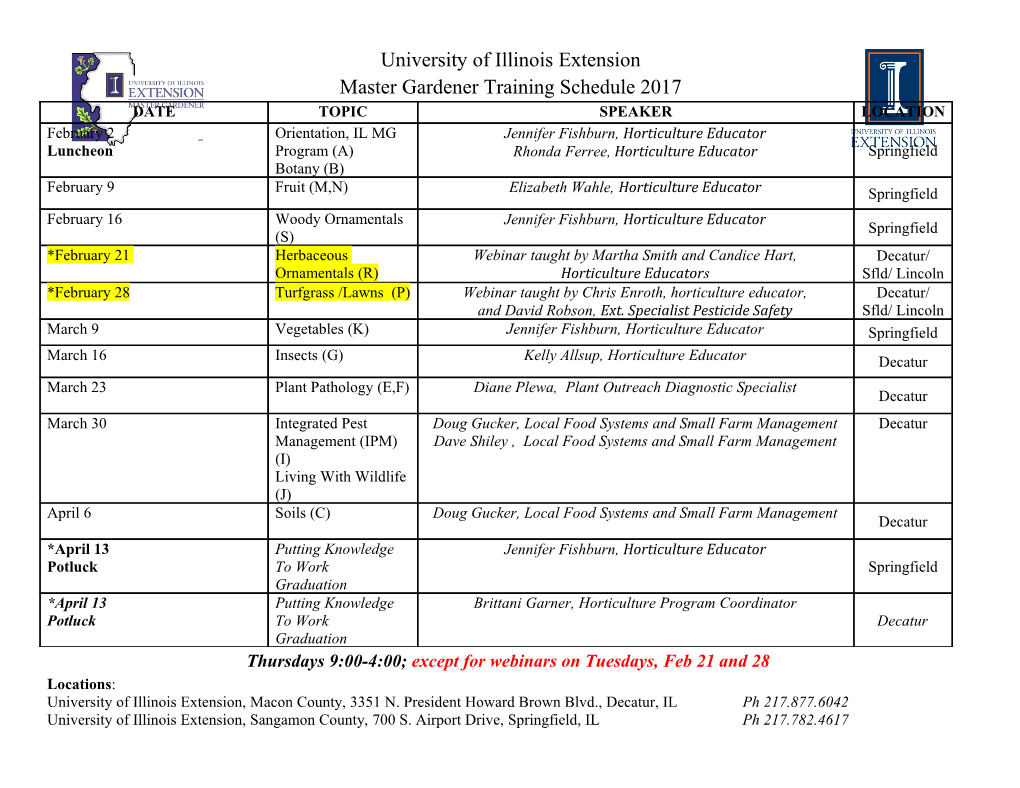
EMERGY SYNTHESIS: Theory and Applications of the Emergy Methodology Proceedings from the First Biennial Emergy Analysis Research Conference, Gainesville, Florida, September, 1999. Edited by Mark T. Brown University ofFlorida Gainesville, Florida Associate Editors Sherry Brandt-Williams Rookery Bay National Estuarine Research Reserve Naples, Florida David Tilley Texas A&M University Kingsville, Teias Sergio U1giati University ofSiena Siena, Italy December, 2000 The Center for Environmental Policy Department of Environmental Engineering Sciences University of Florida Gainesville, FL iii 2 Energy, Emergy and Embodied Exergy: diverging or converging approaches? Sergio Ulgiati ABSTRACT The emergy accountingprocedure is evaluated in light of (i) the need for a clearer statementof its thermodynamicbasis, and (ii) the recent large demandfor integrationamong biophysicalapproaches to investigate natural andhuman-dominated ecosystems. The huge potential of the emergyapproach to catalyze the e./Jorts and improve results of world-wide scientific research in resource use and energy systems assessments is discussed and a code of practice for emergy and biophysical evaluations is suggested INTRODUCTION Concepts underlying biophysical approaches presented in this paper need to be defined and their relationshipshighlighted, in orderto avoid misunderstanding the methodologiesthat areused. The concepts of mass and energy flow are easily understood and theoretically accepted, even if the laws of mass and energy conservation are very often neglected. Some scientists have found it more difficult to accept the two concepts exergy and emergy, mainly due to lack of clear definitions or to unclear presentation of the advantages of their use. Clarifying these two concepts and relating them to other concepts used in thermodynamic and biophysical analyses might help build integrated methodologies.Different approaches may enhance each other, if their use is consistent with the goals of the investigation. Measuring Energy as Heat The energy content of an item may be a misleading concept. Some items (oil, coal, wood, etc.) are usually considered fuels, as they react with oxygen in the air and release a huge amount of heat (the combustion process). Other substances (all food items) also react with oxygen but release heat in a more controlled way within living organisms. They are not considered to be fuels even though they actually "fuel" a metabolic process. Finally, other chemical species and compounds undergo very slow chemical reactions and release a very small amount of heat (for instance, iron oxidation). Every time a resource is transformed, an exchange of energy with the surrounding environment is involved. The energy that is released in the form of heat can be accountedfor as higher heating value, lower heating value, combustion enthalpy, etc., and measured injoules or calories. HHY., the Higher Heating Value, is defined as the total energy contained "in a unit weight or volume offuel and is determinedexperimentally by burninga knownquantity of fuel in a bomb calorimeter and measuring the heat released" (Lyons et aI., 1985). It is sometimes referred to as "gross calorific value", or as "anhydrous gross calorific value" if the fuel has no water content before it is burned.When measuring the heat released, water vapourfrom the products of combustion is condensedin the calorimeter and heat of evaporation/condensation is also accounted for. -15- Chapter 2. Energy, Emergy and Embodied Exergy... Instead L.HY, the Lower Heating Value, assumes that "the moisture products of combustion remain in the vapour phase passing all heat recoverydevices and no condensation heat release is gained' (Lyons et al., 1985). By definition, the L.H.Y, sometimes referred to as "net calorific value", is always lower than the HHY If other heat losses are accounted for in addition to the heat carried away by water vapour, we remain with the U.H. Y, the Usable Heating Value, i.e. "the amount ofheat that can be actually recoveredwhen ajuel is burned in a combustion chamber". It depends, of course, upon the environmental and technical characteristics of the process. If a constant-volume, adiabatic,bomb calorimeteris used, total heat from combustionis accounted for as H.H.Y Instead, if the system is subjected to a constant pressure p, and is allowed to undergo changes of its volume V,then some of the energy supplied as heat may escape back into the surroundings as work. To account for this phenomenon, the concept enthalpy, II, has been introduced, defined as "the heat supplied at constant pressure" (Atkins, 1994): H=U+pV (I) where U is the internal energy of the system. Because combustionis a chemical reaction completely oxidizing a fuel, we may define astandard combustion enthalpy as the total heat that is supplied by the fuel when it is oxidized to CO2 and H20 at constant pressure. The consequence of the above definitions is that it is not sufficient to say "heat of combustion" or "heat content" to clearly indicate the work potential of a resource. The latter depends upon the specific environmental and technical conditions, which must always be stated whenever the process is described. Free-energy and Exergy Because energy may have different ability to provide useful work, depending upon the irreversibility of the process, a more convenient measure can be obtained by means of the concept free-energy.The thermodynamic basis of the free-energy concept is found in the definitionsof Helmholtz and Gibbs energies for systems in thermal equilibrium with their surroundings (Atkins, 1994). When a change in the system occurs and there is a transferof energy as heat betweenthe system and its surroundings, it may occur at constant volume (dU - T dS '" 0) or at constant pressure (dH- T dS" 0), with an entropy change dS= (dU +pdV)lT. In the first case, the Helmholtz free-energy A=U -TS (2) can be introduced; in the second case the Gibbs free-energy G=H-TS (3) is defined'. As most real processes occur at constant pressure, the Gibbs free-energy is far more widely encountered than Helmholtz free-energy, so that G is normally referred to as simply the "free-energy". In bothcases, it can be demonstratedthat a change in free-energy, AG, measures the maximum non-expansion work that the system can do when it is working reversibly (Atkins, 1994). Therefore, free-energy relative to a given reference state can be definedas the maximum amount of work that can be obtained when a system moves froma statecharacterized by a set of parameters {<I>i} (temperature, pressure, chemical potentials, etc.) towards the set of parameters {<Pio} describing the reference state, by means of a reversible process. For chemical and physical processes investigated under laboratory conditions, the reference state is usually characterized by standard laboratory parameters (Atkins, 1994) that are not always equal to those in the environment (e.g., standard temperature is 25°C, while average environmentaltemperature is around 16°C). When the reference state is characterized by average environmental values of parameters, free-energy is usually called exergy (Szrugut et al, 1988), to make it clear that we refer to the surrounding environment. According to Szargut et al. (1988), "exergy is the amount ofwork obloinablewhen some matter is brought to a slaleof thermodynamic equilibrium with the common componentsof the naturalsurrou ndings by means of reversibleprocesses, involving interactiononly with the above mentionedcomponents of nature." , u- internal energy; T - temperature; p = pressure; V = Volume; S = entropy; H = enthalpy; dS, dV, dU, and dH areinfinitesimalchanges ofvariables -16- Chapter 2. Energy, Emergy and Embodied Exergy... Except for a few cases such as electricityproduction by means of nuclear reactors, the gravitational potential of water or the geothermal potential of deep heat, chemical exergy is the only significant free-energy source in most processes. Inthe procedureof Szargut et al. (1988), chemical exergy is calculated as the Gibbs free-energy relative to average physical and chemical parameters of the environment. Szargut et aJ. (1988) calculated the chemical exergy of a resource, bch,res ' relative to common components of nature, by means of the following procedure: (a) The most stable end products of reactions involving the resource under consideration are chosen as reference chemical species "rs" in the environment (air, ocean or earth crust). These reference species are not in thermodynamic equilibrium, as the biosphere is not a thermodynamic equilibrium system. Reference species are assigned a specific free-energy (exergy) brs due to their molar fraction Zrs in the environment by means of the formula = brs - RT In Zrs where the reference species are assumedto form an ideal mixture or solution, R is the gas constant, T is the environment temperature. (b)In accord with the classical procedure of Gibbs, a chemical reaction linking a resource "res" to its reference species is chosen. The chemical exergy difference Abcb,res between the two = � states is calculated as Abch,res Abf+ bch i where Abfis the standard chemical exergy of formation of the resource from elements, and bch i is the standard chemical exergy of the i-th element relative to its reference species. Tabulated values (Atkins, 1994) of free-energy can be used for this purpose. bch,res = Abf+ � +� (c) The total chemical exergy of the resource is finally calculated as bch i brs' In so doing, tables of exergies per urtit mass or per mole (specific
Details
-
File Typepdf
-
Upload Time-
-
Content LanguagesEnglish
-
Upload UserAnonymous/Not logged-in
-
File Pages18 Page
-
File Size-