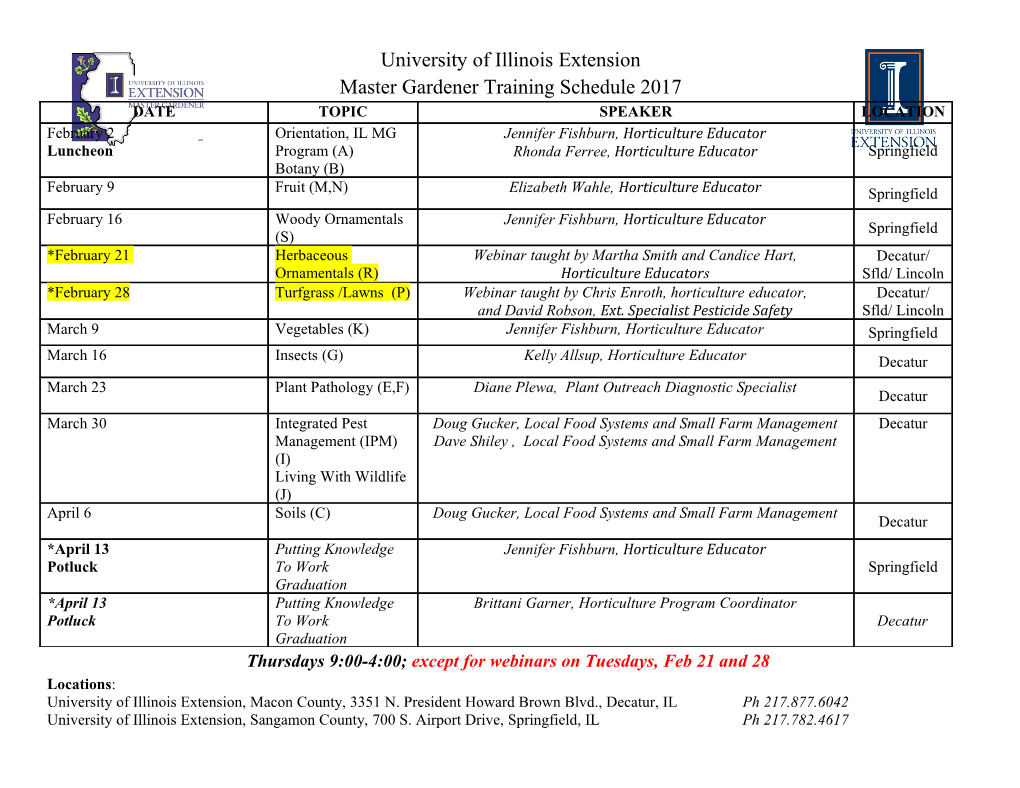
2. Review of Ion Implantation 2.1. Ion implantation of polymers 2.1.1. Polymer structure The term “polymer” describes an extremely diverse group of materials, defined by their being composed of high-molecular weight molecules. Further distinction from other groups of materials is difficult since polymers may be amorphous or crystalline, liquid or solid, organic or inorganic, conductive or insulating. The largest category of polymers, and the one which shall be dealt with in this work, is carbon based, formed by polymerisation of organic precursors. They may be described as amorphous agglomerations of long carbon chains, or backbones, with functional or passive side- groups attached to the carbon atoms. Average molecular masses of individual macromolecules in polymers range from 104 to 106 u. Although predominantly amorphous, crystalline domains exist within semicrystalline polymers and some polymers are completely crystalline. The simplest of the organic polymers is polyethylene, formed by polymerisation of ethylene (CH2CH2) into polymer molecules many thousands of repeating units long. It may be described by [-CH2CH2-]n, where n is a number usually greater than ten thousand. Bulk properties of the material are dependent on the length of the polymer chains. Increasing melting temperature is exhibited by low molecular weight (or low density) polyethylene (LDPE), medium density polyethylene (MDPE) and high 4 density or ultra-high molecular weight polyethylene (UHMWPE) respectively. Substitution of one or more of the hydrogen side-groups by using a different precursor gas results in variants with distinctive bulk properties. For example, substitution of hydrogen for a methyl group gives polypropylene, for a cyano group gives polyacrylonitrile (Acrylic), and for chlorine gives poly(vinyl chloride) (PVC). Substitution of all four hydrogen atoms for fluorine results in poly(tetrafluoroethylene) or PTFE, trade name “Teflon”. Substitution of carbon atoms in the backbone with oxygen, nitrogen, sulphur and carbon ring structures, and combinations of polymer unit sequences, termed co-polymers, provides almost unlimited variation in structure, and subsequent physical and chemical properties An important concept when considering the physical state of a polymer is the notion of a glass transition temperature (Tg). The glass transition temperature for some common polymers is given in table 2.1. Cooling of a polymer melt decreases the vibrational energy of the constituent molecules. Due to strong long-range intermolecular forces, the transition from the liquid to the solid state occurs over a large temperature range. The glass transition temperature is assigned according to readily observable changes in the thermodynamic properties of the polymer. For example, the heat capacity and expansivity change discontinuously at the glass transition temperature. Mechanical properties also change rapidly. At room temperature, poly(methyl methacrylate) (PMMA) is below its Tg and is relatively hard and brittle, whilst above its Tg PMMA is soft and rubbery. The degree of crystallinity of a polymer is affected by the rate of cooling through the glass transition temperature. Rapid quenching of a polymer melt results in a highly amorphous solid phase, whilst slow cooling encourages the growth of microcystalline domains. 5 Table 2.1: Some common polymers, their repeating units, chemical structure and glass transition temperatures Name Repeating unit Chemical structure Tg (°K) Polyethylene [-CH2CH2-]n 140-270 Poly(vinyl [-CH2CH(Cl)-]n 354 chloride) Polystyrene [-CH2CH(C6H5)-]n 373 Polypropylene [-CH2CH(CH3)-]n 250 Polycarbonate [-OC6H4C(CH3)2 421 C6H4OC(O)-]n Poly(tetrafluoro- [-CF2CF2-]n N/A ethylene) Bulk properties of polymers are as varied as the chemical structures of which they are composed. In general, polymers exhibit relatively low density when compared to other classes of solid. This is due to the random arrangement of large molecules, resulting in large areas of empty space. Chemical inertness, especially resistance to attack by acids, is another common property of polymers. Whilst extremely useful in 6 some respects, this property limits the applicability of polymers to many conventional processing techniques. For example, adhesion of metal films to polymer surfaces is an area of great interest for applications in microelectronics. Traditional metallisation processes often result in low film adhesion due to lack of interaction between the film and the substrate. Plasma processing of the surface prior to deposition by plasma condensation results in an improved adhesion [1]. Plasma processing and ion implantation are non-contact processes that have successfully been applied for surface modifications of polymers. A review and discussion follows. 2.1.2. Ion-polymer interactions Low-energy plasma treatments (ion energy < 100eV), such as exposure of a polymer surface to DC or RF glow discharge plasmas, affect only the near surface regions. Impacting low-energy ions can break covalent bonds at the surface, exposing functional groups that affect the surface energy, and subsequently modify the surface hydrophobicity [2]. Chemically reactive ions can become incorporated into the surface chemical structure, functionalising the surface for specific interactions with molecules, proteins and cells [3]. High doses of low energy ions can adsorb on or near the surface, congregating together to form islands, eventually resulting in thin film growth. Conventional ion implantation uses a series of high voltage grids to extract ions from a plasma and accelerate them as an ion beam toward a target material in a low- pressure environment. Medium- (100eV-100keV) and high-energy (>100keV) ions implant beneath the surface, affecting the target material in a number of different 7 ways. Since polymers are a relatively soft material, composed of, on average, 20% free space, implanting ions travel a comparatively large distance before coming to rest. In the absence of thermodynamic constraints present in traditional materials processing, introduction of a foreign species into the target material can form new compounds. High doses into metals can form new alloys or ceramics. Foreign species act as dopants in semiconductors, which is currently the primary commercial application of ion implantation. High doses of metal ions in polymers can increase the conductivity by forming conductive networks. A more important consideration for polymers, than the presence of foreign ions or atoms, is the “damage” to the polymer molecules that the ions cause before they come to rest. Impacting medium- to high-energy ions lose energy by multiple interactions with the target material. A convenient measure of the energy deposited by an ion along its path inside the target material is the linear energy transfer (LET), often expressed in units of eV/nm. Interactions can be classified into two categories; interactions of the ion with the target nuclei are known as nuclear stopping processes, and interaction with target electrons are known as electronic stopping processes. Electronic (Se) and nuclear (Sn) stopping powers (measured in LET) can be calculated by considering the interaction cross-sections as a function of incident ion species, ion energy and target atoms. Electronic stopping occurs by two processes, both involving electromagnetic interaction between the positively charged ion and target electrons. Relatively frequent low-energy-transfer inelastic glancing collisions usually result in excitation of orbital electrons to a higher energy level (plasmons). High-energy-transfer elastic 8 knock-on collisions, whilst more infrequent, transfer a much greater momentum to the target electrons and are more likely to result in ionisation. Ionisation results in the creation of a molecular ion and the liberation of secondary electrons, which either exit the target material or relocate to another region in the target. Nuclear stopping involves the transfer of momentum from the ion to a host matrix atom, or recoil atom. If the momentum transfer is small the energy imparted to the recoil atom will be dissipated by phonons. Higher energy transfer collisions may result in irreversible displacement of the recoil atom from its original site. This process requires enough energy to break the bonds that held the atom in place. The displaced atom may remain bound to other atoms or dissociate completely. Displaced recoils are capable of creating further recoils and ionisation. Calculation of the total Sn by an incident ion must include the contribution from recoils as well as the primary ion. Increasing the ion atomic number linearly increases the nuclear interaction cross- section, subsequently increasing the recoil displacement probability. A Monte-Carlo simulation program, TRIM 2003 (Transport of Ions in Matter) [4] calculates the Se and Sn for a given ion species, energy and target material. An example of electronic and nuclear stopping energy as a function of target depth for 10kV argon ions into PMMA (parameters typical of the experiments presented in this thesis) is shown in figure 2.1 for both the incident ion and recoil ions, averaged for 100,000 ions. The sum of the total energy under the six curves equals the 10kV of the incident ion. Electronic stopping is highest near the surface when the ion energy is highest. Incident ion nuclear stopping shows a distinct peak at a depth where the ion energy is reduced to a value corresponding to the maximum interaction cross-section. 9 This is because nuclear
Details
-
File Typepdf
-
Upload Time-
-
Content LanguagesEnglish
-
Upload UserAnonymous/Not logged-in
-
File Pages35 Page
-
File Size-