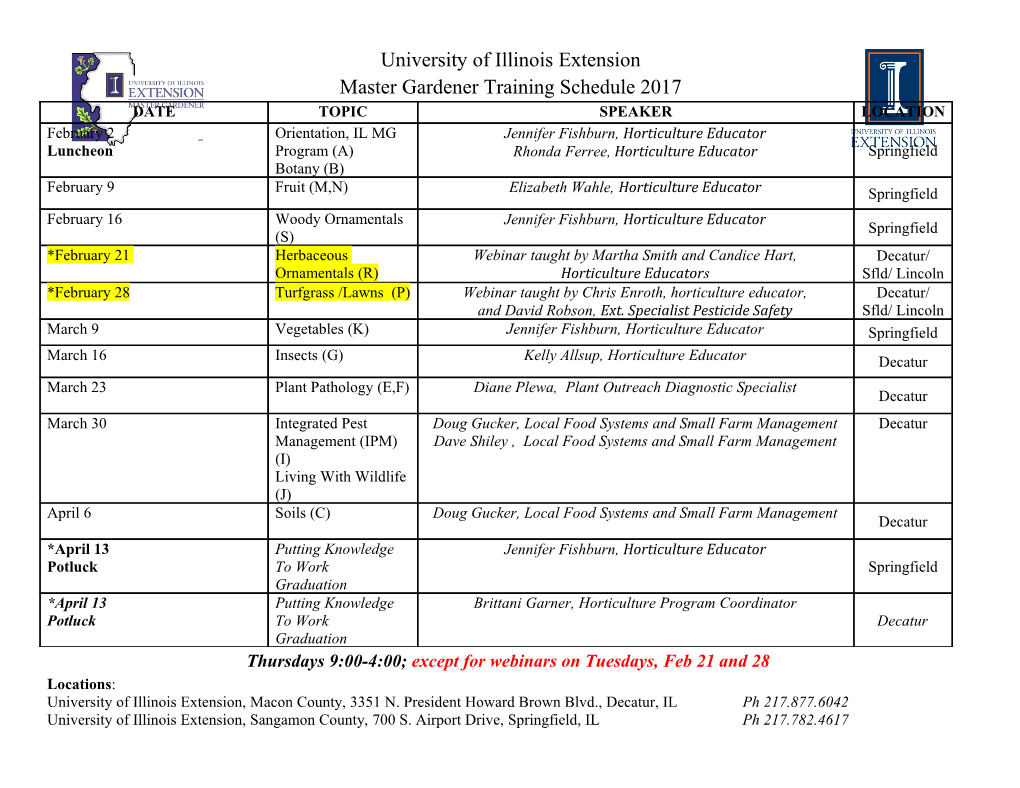
WDS'17 Proceedings of Contributed Papers — Physics, 116–120, 2017. ISBN 978-80-7378-356-3 © MATFYZPRESS Investigation of Ion Interaction with Glyoxal M. Lacko,1,2 F. Piel,3,4 and P. Španěl1 1 J. Heyrovsky Institute of Physical Chemistry of the CAS, v. v. i, Dolejškova 2155/3, 182 23 Prague 8, Czech Republic. 2 Charles University, Faculty of Mathematics and Physics, Prague, Czech Republic. 3 IONICON Analytik GmbH, Eduard-Bodem-Gasse 3, 6020 Innsbruck, Austria. 4 Institut für Ionenphysik und Angewandte Physik, Leopold-Franzens-Universität Innsbruck, 6020 Innsbruck, Austria. Abstract. Glyoxal is an unstable and extremely reactive molecule represents an important part of biological processes. The detection of glyoxal using soft chemical ionization techniques is, due to its behavior, nontrivial. In this article we present + + + results of investigation of the reactions of thermalized H3O , NO and O2 ions with glyoxal molecules interactions using SIFT-MS experimental technique. The main result is characterization of the dependences of these reactions on variable sample humidity corresponding to flow tube H2O concentrations. We have observed + formation of protonated formaldehyde (m/z 31) from H3O reagent ions in presence of + glyoxal and water. Additionally, association into M(NO) ion and charge transfer into +. + +. M ion was observer for NO and O2 ions respectively. Introduction Glyoxal (C2H2O2, Ethane-1,2-dione, CAS: 107-22-2) is the simplest dialdehyde. This molecule is highly reactive especially in humid environments and the presence of glyoxile is associated with some basic biological processes. Glyoxal was associated as a source of oxidation stress [Manini et al., 2015] or as a part of catalytic reaction formed basic organic molecules in inorganic environment [Shkrob et al., 2012]. Experimental investigation using SCI (Soft Chemical Ionization) techniques was often predicting formation of protonated glyoxal with m/z 59 [Williams et al., 2001, Knighton et al., 2007] as a result of proton transfer process with + H3O reagent ion, which can be used for evaluation of glyoxal presence in environment. Previous PTR-MS study [Stönner et al., 2017] showed significant dependence of abundance of the product with m/z 59 on water vapor concentration. Moreover, transformation of the product with m/z 59 into a product with m/z 31 was observed, correlating with increasing water vapor concentration of the system, associated with + formation of protonated formaldehyde (CH2O⋅H ). Authors excluded potential water–glyoxal reaction resulting to the production of formaldehyde in the inlet system using absorption spectrometry of the inlet system. Mechanism of protonated formaldehyde formation out from glyoxal was not discussed. Proton affinity (PA) of glyoxal was investigated only theoretically by Wróblewski et al. [2007] using HF and B3-LYP methods with 6-311G** split-valence molecular orbitals basis sets. Authors obtained good agreement of PA for calculated organic molecules compare to available experimental data, especially for B3- LYP method with maximal relative difference 5 kcal/mol (0.84 kcal/mol for acetone, 1.19 kcal/mol for formaldehyde, anyway ≈ 7 kcal/mol for ketene). Without experimental data, PA was estimated with values 165.06 kcal/mol and 161.41 kcal/mol for HF and B3-LYP calculation respectively. Proton affinity of water (165.15 kcal/mol) [NIST 2017] is similar to the calculated PA of glyoxal. Due to this proton transfer should occur only at very slow rate constants/should not be very efficient, eventually with significant amount of backwards reactions. In present study we investigated interaction of glyoxal molecule with three reagent ions + + +. (H3O , NO and O2 ) using SIFT-MS experiment at variable water vapor concentration. Materials Glyoxal crystals were prepared from 40% glyoxal solution obtained from Sigma-Aldrich Co. Synthesis was based on methylglyoxal preparation of Talukdar et al. [2011] visualized in Figure 1. Approximately 10 ml of glyoxal solution was placed into 250 ml flask and pumped down using membrane pump for 16 hours to remove water. Expected high viscose residuum in our case has transformed into white crystals formed by polyglyoxals. Crystals were subsequently covered by separation layer of glass bullets followed by phosphorous pentoxide layer to remove remaining water vapors. Heating the system up to app. (150–200) °C provided thermal decomposition of polyglyoxals was applied until yellow vapors characteristic for glyoxal appeared. Gas phase glyoxal crystalized into a cold trap cooled by dry ice. Manipulation with the sample was provided inside a box with dry ice forming CO atmosphere to reduce sample exposition to the atmospheric water vapor concentration. 116 LACKO ET AL.: INVESTIGATION OF ION INTERACTION WITH GLYOXAL Figure 1. Chemical setup for synthesis of pure glyoxal crystals. Methods + + +. Investigation of glyoxal interaction with H3O , NO and O2 reagent ions was carried out using a SIFT-MS experiment connected to a precise gas standards generator (PGSG) unit presented in Figure 2. PGSG provided a controlled concentration of glyoxal. In presented investigation we used dry air as a dilution gas, supplemented by small water reservoir to control water vapor concentration level of dilution gas. Water vapor concentration was variated by water evaporation controlled by heating or cooling of the water reservoir using a hand heater or a dry ice in the temperature scale from –78.5 °C to ≈ 50 °C. Solid state sample was introduced into a small 2 ml glass bottle closed with septa penetrated by 5 cm long capillary (I.D. 0.25 mm) made from PEEK (Polyether ether ketone) and placed into a heated (50 °C) sampling area of the PGSG unit. The SIFT-MS experiments [Smith et al., 1999] were carried out using a Profile 3 (Instrument Science, Crewe, UK) instrument. Reagent ions are generated using microwave discharge in water vapor, see Figure 2. Ions are subsequently focused into a quadrupole mass spectrometer, where they are selected as defined reagent + + +. ions (H3O , NO or O2 ). The selected ions are then focused into a 5 cm long flow tube and injected into a helium carrier gas (pressure p = 1.4 mbar, temperature T = 24 °C), where they are thermalized. Air samples are introduced into the carrier gas at a known flow rate, where they interact with precursor ions. Interaction time is defined by the length of the flow tube and by the flow rate of helium medium. The product ions generated/created by interaction are then introduced into a quadrupole mass analyzer. There they are separated according to their m/z and afterwards detected by a secondary electron multiplier (SEM). Water vapor concentration of sample was changed from room temperature into –78.5 °C by putting water container into a dry ice. After temperature stabilization, water container was removed from the dry ice and gently heated up to ≈ 50 °C. Continuous change of water vapor concentration together with product ion evolution was monitored using continuous measurement of selected m/z abundances. Moreover, during the evolution we were + + +. periodically switching between H3O , NO and O2 primary ions, 60 s for each, to provide continuous investigation of all three reagent ions. Level of water vapor concentration was estimated as a logarithm of relative ratio of H O+ reagent ion to higher water clusters: . 3 [ ] [ ] +[ ( ) ] �퐻3푂 � + + + −푙푙 � 퐻3푂 + 퐻3푂 ∙퐻2푂 + 퐻3푂 ∙ 퐻2푂 2 + ⋯� Results The profile of water vapor concentration change during the experiment is presented in Figure 3. Water + +. vapor concentration values for NO and O2 ions were estimated using linear interpolation of water vapor + concentration level between successive H3O measurements. For all reagent ions several ions were tracked, namely: m/z 31, m/z 43, m/z 57, m/z 58, m/z 59, m/z 76, m/z 77, m/z 88, m/z 94, m/z 95, m/z 106, representing potentially products of association, ionization or dissociation. + Interaction of glyoxal with H3O reagent ion is presented in Figure 3b and schematically described in Scheme 1. For low water concentration, protonated glyoxal is formed. With increasing water vapor concentration + + level, formation of MH (H2O) and MH (H2O)2 clusters were observed, however with low abundance even for 117 LACKO ET AL.: INVESTIGATION OF ION INTERACTION WITH GLYOXAL Figure 2. Experimental configuration used for ion-molecular interaction investigation. Dry air is introduced into the PGSG directly or through a water container. Water vapor concentration of input air is regulated by temperature of water reservoir. Glyoxal concentration is regulated by heating the sample up to the 50 °C followed and dilution of vapors with introduced air. Generated mixture is then introduced into the SIFT-MS instrument. high water concentration levels. Formation of m/z 31 fragment associated with production of protonated + formaldehyde (H2CO⋅H ) was observed as well with clear dependence on increasing water concentrations. Interaction of glyoxal with NO+ reagent ion leads to association process formed product with m/z 88 represented as M(NO)+ ion (1) Abundance of the signal strength normalized to total primary ion current is presented in Figure 3c. Except formation of M(NO)+ ion, formation of the glyoxal cation was detected as well. Both ions are weakly dependent on water vapor concentration variation with decreasing trend. Similar decrease of signal was observed for +. formation of the ionized glyoxal as a result of charge transfer using O2 reagent ion (Figure 3d) (2) Effect of the signal strength loss may represent decreasing efficiency of glyoxal sublimation from solid state or polymerization and followed deposition of glyoxal vapors interacting with water molecules. + +. For both NO and O2 reagent ions we observed formation of ion with m/z 57, potentially represented by a hydride abstraction of glyoxal (M–H)+. Product is significantly contributing into fragmentation pattern at low water vapor concentrations, followed by decrease of abundance and repeatedly by increase for high water vapor + concentration. Observation confirmed higher abundance of ion with m/z 57 for O2 reagent ion with respect to M+ ion formation, compare to opposite trend for NO+ reagent ion.
Details
-
File Typepdf
-
Upload Time-
-
Content LanguagesEnglish
-
Upload UserAnonymous/Not logged-in
-
File Pages5 Page
-
File Size-