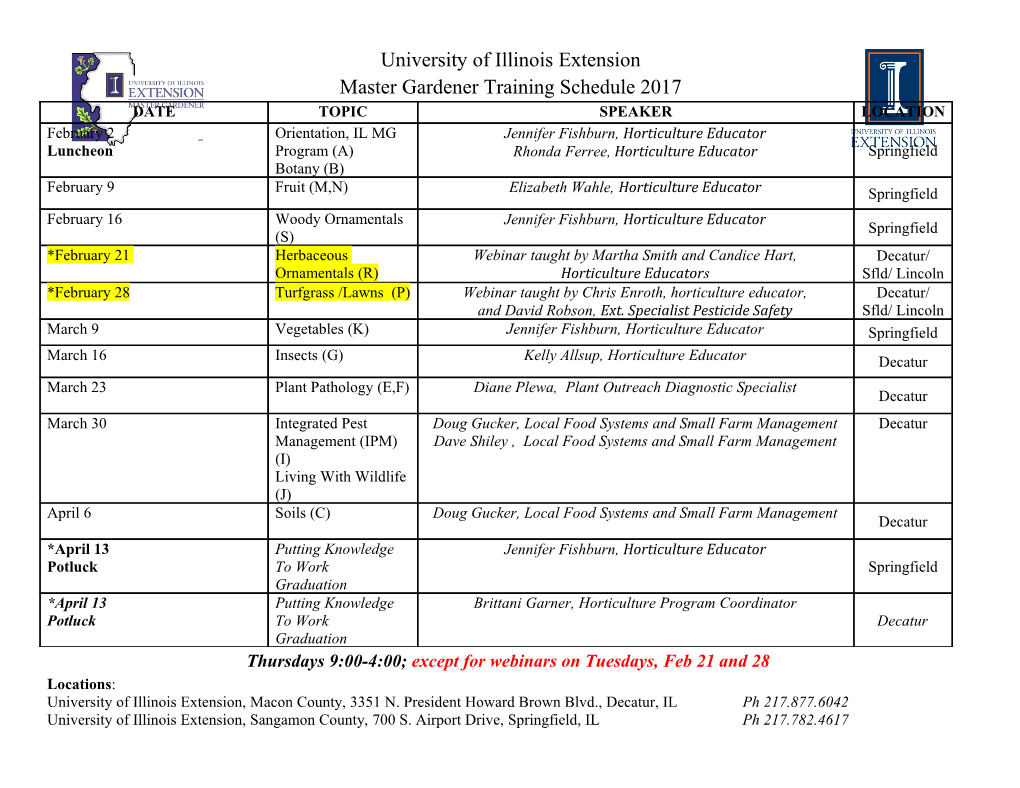
Eur. Phys. J. A manuscript No. (will be inserted by the editor) Light dark matter searches with positrons M. Battaglieri1,2, A. Bianconi3,4, P. Bisio5, M. Bondì1, A. Celentano1, G. Costantini3,4, P.L. Cole6, L. Darmé7, R. De Vita1, A. D’Angelo8,9, M. De Napoli10, L. El Fassi11, V. Kozhuharov7,12, A. Italiano10, G. Krnjaic13,14, L. Lanza8, M. Leali3,4, L. Marsicano1,a, V. Mascagna4,15, S. Migliorati3,4, E. Nardi7, M. Raggi16,17,a, N. Randazzo10, E. Santopinto1, E. Smith2, M. Spreafico5, S. Stepanyan2, M. Ungaro2, P. Valente17, L. Venturelli3,4, M.H. Wood18 1Istituto Nazionale di Fisica Nucleare, Sezione di Genova, 16146 Genova, Italy 2Thomas Jefferson National Accelerator Facility, Newport News, Virginia 23606 3Università degli Studi di Brescia, 25123 Brescia, Italy 4INFN, Sezione di Pavia, 27100 Pavia, Italy 5Università degli Studi di Genova, 16146 Genova, Italy 6Lamar University, 4400 MLK Blvd, PO Box 10046, Beaumont, Texas 77710 7Istituto Nazionale di Fisica Nucleare, Laboratori Nazionali di Frascati, Via E. Fermi 54, Frascati, Italy 8INFN, Sezione di Roma Tor Vergata, 00133 Rome, Italy 9Università di Roma Tor Vergata, 00133 Rome Italy 10Istituto Nazionale di Fisica Nucleare, Sezione di Catania, 95125 Catania, Italy 11Mississippi State University, Mississippi State, Mississippi 39762-5167, USA 12Faculty of physics, University of Sofia, 5 J. Bourchier Blvd., 1164 Sofia, Bulgaria 13Fermi National Accelerator Laboratory, Batavia, Illinois 60510, USA 14Kavli Institute for Cosmological Physics, University of Chicago, Chicago, Illinois 60637, USA 15Università degli Studi dell’Insubria, 22100 Como, Italy 16Sapienza Università di Roma, piazzale Aldo Moro 5 Roma, Italy 17Istituto Nazionale di Fisica Nucleare, Sezione di Roma, piazzale Aldo Moro 5 Roma, Italy 18Canisius College, Buffalo, NY 14208, USA Draft : May 27, 2021 Abstract We discuss two complementary strategies to 1 Introduction and motivations search for light dark matter (LDM) exploiting the posi- tron beam possibly available in the future at Jefferson One of the most compelling arguments motivating the Laboratory. LDM is a new compelling hypothesis that search for extensions of the Standard Model (SM) is identifies dark matter with new sub-GeV “hidden sec- the need to explain the nature of dark matter (DM). In tor” states, neutral under standard model interactions years past, theoretical and experimental efforts mainly and interacting with our world through a new force. catalysed around the hypothesis that DM corresponds Accelerator-based searches at the intensity frontier are to a Weakly Interacting Massive Particle (WIMP) with uniquely suited to explore it. Thanks to the high inten- electroweak scale mass. Such a hypothesis is well ground- sity and the high energy of the CEBAF (Continuous ed since in the early Universe WIMPs would be pro- Electron Beam Accelerator Facility) beam, and relying duced via thermal processes, and their annihilation with arXiv:2105.04540v2 [hep-ex] 25 May 2021 on a novel LDM production mechanism via positron typical weak interaction rates would leave, almost inde- annihilation on target atomic electrons, the proposed pendently of other details, a relic density of the correct strategies will allow us to explore new regions in the size to explain DM observations. However, despite an LDM parameters space, thoroughly probing the LDM extensive search program that combined direct, indi- hypothesis as well as more general hidden sector sce- rect, and collider probes, to date no conclusive signal narios. of the WIMP has been found [1]. This prompts the sci- entific community to put no lesser vigor in exploring alternative pathways. Feebly interacting particles (FIPs) represent a par- aContact authors: [email protected], ticularly interesting alternative to WIMPs. In recent [email protected] years the physics of FIPs has focused a steadily growing 2 interest, as characterised witnessed by the remarkable nised that this process is of more general importance number of community reports and white papers that since, due to the presence of secondary positrons in have appeared in the last few years [2,3,4,5,6,7]. FIPs EM showers, it contributes to FIPs production also are exotic and relatively light particles, not charged un- in electron [18,19] and proton [20] beam experiments, der the SM gauge group, whose interactions with the it is clear that the availability of a beam of primary SM fields are extremely suppressed. FIPs are often as- positrons is of utmost importance to fully take advan- sumed to be part of a possibly complicated secluded tage of the resonant production channel. sector, called the dark sector, with the Lightest stable dark particle(s) playing the role of DM (LDM). This scenario has sound theoretical motivations: in first place 1.1 Dark sectors and relic density targets many known particles are uncharged under some gauge group factors, so that the existence of particles blind Experimental proposals aiming at detecting missing en- ergy from the apparatus are mostly sensitive to the to all SU(3)C SU(2)L U(1)Y interactions seems a rather natural× possibility.× Secondly, theoretical mech- FIPs nature and couplings. While this allows such search anisms like gauge symmetries or quasi-exact sponta- strategies to cover a broad class of new light physics neously broken global symmetries, that we know are models, the details of the dark sector to which the DM realised in Nature, can explain why some particles re- belongs remain ultimately inaccessible. Relic density main light even when they are associated with physics targets thus must rely on additional theoretical assump- at some large scale. From the phenomenological point of tions. Arguably the most elegant models for LDM are view, light weakly coupled new particles have been often those which reproduce the successes of WIMP models, invoked to account for several discrepancies observed being: UV-insensitive, predictive and as economical as in low energy experiments. Examples are the measured possible. A generic expectation is then that DM anni- value of the muon anomalous magnetic moment [8], the hilation proceeds via a bosonic feebly-interacting medi- proton charge radius measured in muonic atoms [9,10, ator, taking care that by the time of CMB annihilation 11], the discrepancy between neutron lifetime measure- is sufficiently suppressed to evade standard limits on ments in bottle and beam experiments [12,13], the mea- energy injection [21,22,23]. sured abundance of primordial 7Li which is a factor of Various simplified structures for the dark sector it- three lower than BBN predictions [14], the ‘bump’ in self have been considered throughout the years, ranging the angular distribution of e± pairs observed by the from basic scenarios such as a complex scalar DM to Atomki collaboration in nuclear decays of 8Be [15] and more advanced setups as asymmetric DM or inelastic 4He [16] excited states. DM with an additional dark Higgs boson. The broad 2 target region where ΩDMh = 0:11933 0:00091 [23] FIPs scenarios hint to a remarkably broad range corresponds to mediator FIPs in the 1 MeV± 10 GeV of possibilities, ranging from the very nature of the mass window with coupling suppressed as 10−5 10−2 new particles (scalars, pseudoscalars, fermions, spin- respectively. However, the precise relic density− target one bosons) and spanning several order of magnitude strongly depends on the details of the model. Assum- in mass and couplings. To thoroughly explore all these ing for simplicity a dark photon A0 scenario, interaction possibilities will require an extensive collaboration among with the SM can proceed via a kinetic mixing ". The a variety of small/medium scale experiments, exploit- dark photon couples with the electromagnetic current ing diversified detection techniques, and operating at µ em with coupling e" and with the dark gauge current different facilities. Accelerator-based searches exploit- J µ leading to: ing positron beams stand out as a particularly pow- JD 0 µ 0 µ erful tool. This is because for any given beam energy A µe" em A µgD D : (1) L ⊃ − J − J there is a range of masses where dark bosons can be Different DM candidates corresponds to different choices produced resonantly through positron annihilation on of dark currents (see e.g. a summary in [24]) and differ- atomic electrons in the target, yielding a huge enhance- ent thermal targets: ment in the production rate. As it was first pointed out 8 in Ref. [17], for resonant production there is no need i (χ∗@µχ χ∂µχ∗) (Complex Scalar DM; χ) > that the beam energy will be tuned to match in the <> − µ = 1 µ 5 c.m. the precise value of the mediator mass. This is be- D 2 χγ¯ γ χ (Majorana DM; χ) J > cause due to the continue loss of energy from soft pho- > µ :iχ¯1γ χ2 (pseudo Dirac DM; χ1) : ton bremsstrahlung, in the first few radiation lengths − of a dump a positron beam can continuously scan for In the simplest cases where the DM relic density is fixed production of new resonances. While it was later recog- completely via the freeze-out of a dark photon-induced 3 s-channel annihilation, the final relic density depends A′ only on one variable that, for mχ mA0 , reads: e+ e+ − − 4 2 mχ y " αD : (2) (a) γ ≡ mA0 Depending on the DM nature the typical values of y Z Z required to match the relic density target can vary by e+ A a few orders of magnitude. A simple example of the ef- ′ fect of the dark sector structure on the relic density is (b) found in the inelastic DM scenario (see [25,26] and sub- γ sequent literature). The dominant annihilation chan- e− 0∗ + − nel at low masses, χ1χ2 A e e , depends on + the mass splitting between! the states! (co-annihilation e A′ mechanism [27,28]) as well as on whether the media- (c) tor can be produced resonantly when mχ1 + mχ2 .
Details
-
File Typepdf
-
Upload Time-
-
Content LanguagesEnglish
-
Upload UserAnonymous/Not logged-in
-
File Pages11 Page
-
File Size-