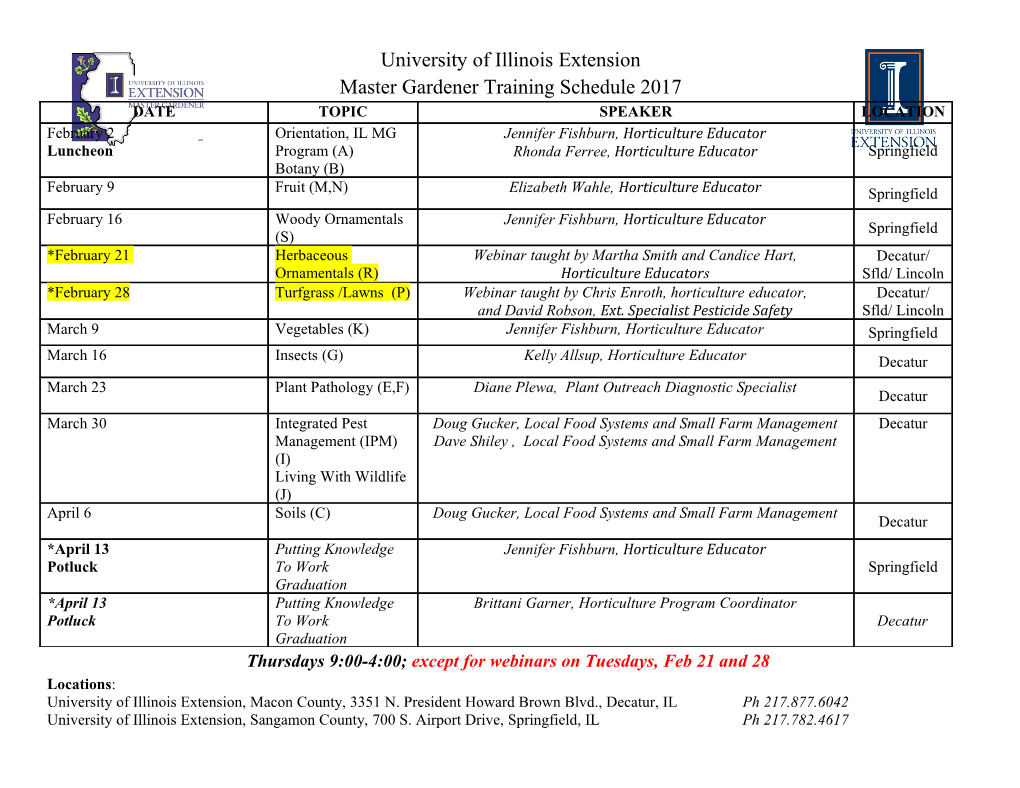
Marine and River Dune Dynamics – MARID VI – 1-3 April 2019 - Bremen, Germany Bedform successions formed by submerged plane-wall jet flows Jörg Lang Leibniz Universität Hannover, Institut für Geologie, Hannover, Germany – [email protected] hannover.de Juan Fedele ExxonMobil Upstream Research Company, Spring, Texas, USA [email protected] David Hoyal ExxonMobil Upstream Research Company, Spring, Texas, USA [email protected] ABSTRACT: Jet flows, expanding from an orifice into a standing water body, are considered as scale-independent model for clastic depositional processes. We conducted 3D experiments with Froude supercritical jet flows, systematically varying several of the controlling parameters such as bed slope, grain size, and flow variables that define the initial densimetric Froude number. The ini- tial inertia-driven jets evolved into gravity-driven flows by rapid expansion and deceleration, there- by forming scours, mouth bars and trains of bedforms. Hydraulic jumps were absent in the flow transition. The geometries of the mouth bar and bedforms were primarily controlled by the initial densimetric Froude number. Further control was exerted by the bed slope, sediment supply and grain sizes. Gravity-controlled processes rapidly take-over the control on the morphodynamic evolu- tion of the flow and are responsible for deposition on the lee side of the mouth bar and beyond. et al., 2003). Jet flows and their deposits 1. INTRODUCTION display a distinct proximal to distal zona- Jet flows are flows that emerge from an tion. Jet-flow deposits are generally charac- orifice into a standing water body and decel- terized by a mouth bar that develops down- erate and expand due to the entrainment of flow of a scour. The downflow facies tracts ambient water. They can be considered as a reflect the deceleration of the flow and pass basic model for clastic depositional process- from a region of by-pass and erosion via a es independent of scale and environment, for region of bedform formation into a region of example deltas (Bates, 1953), submarine suspension settling (Powell, 1990; Hoyal et fans (Beaubouef et al., 2003; Hoyal et al., al., 2003; Russell & Arnott, 2003; Winse- 2003; Terlaky et al., 2016) and subaqueous mann et al., 2009). After the transition from ice-contact fans (Powell, 1990; Russell & an inertia-driven jet flow into a gravity- Arnott, 2003; Winsemann et al., 2009). The driven density flow, the density flow may evolution from inertia-driven jet flows into either evolve into an underflow or rise as a gravity-driven density flows is primarily buoyant plume, depending on the density controlled by the initial momentum and the difference to the ambient water (Powell, density difference between the flow and the 1990). ambient water (Powell, 1990; Hoyal et al., 2003). The rates of jet flow deceleration and 2. 2 METHODS expansion and the expansion angle are pri- marily controlled by the orifice densimetric We conducted 3D tank experiments with Froude number (Fr’). Secondary controlling submerged plane-wall jet flows in an exper- factors for jet flows and their deposits in- imental domain, which comprised an 8 m clude discharge, sediment concentration and long and 5 m wide plate placed in a 10 m grain size (Bates, 1953; Powell, 1990; Hoyal long, 7 m wide and 2 m deep glass-walled tank (Fig. 1A). The evolving flows and de- 151 Marine and River Dune Dynamics – MARID VI – 1-3 April 2019 - Bremen, Germany Figure 1. Experimental jet flows and their deposits. A) Experimental set-up for jet flows. A leveled sediment bed is prepared on the plate. Flows are released from the inlet pipe. B) The expanding jet flow forms a scour and a mouth bar (densimetric Froude number Fr’=4). Flows passing over the mouth bar have already evolved into gravity-controlled density flows. C) Sour and mouth bar of a experimental jet flow (Fr’=2). D) Cross-section of a jet-flow deposit (Fr’=4). posits were documented by photographs ble beds, and (iii) aggrading jet flows on through the tank walls. Velocity (point ADV erodible beds. and profiler) and density (conductivity) probes enabled the collection of flow data during the experiments. After the experi- 3. 3 RESULTS ments the tank was drained and the external The initial inertia-driven jet flows and internal geometry of the deposits was evolved into gravity-driven density flows by measured. rapid flow expansion and deceleration (Fig. The controlling parameters were system- 1B). The turbulent jet flows rapidly expand- atically varied to test their impact on the ed from the orifice by the entrainment of flow dynamics and the resulting deposits. ambient water into large turbulent eddies These controlling parameters include bed that develop at the flow interface. Hydraulic slope, sediment-grain size and the flow vari- jumps were never observed in the expanding ables (discharge, density difference and pipe Froude supercritical jet flow or at the transi- diameter) that define the initial densimetric tion into a density flow. The transition from Froude number. The tested experimental jet to density flow was observed to occur at conditions were classified as follows: (i) a short distance from the orifice, typically at non-aggrading jet flows on non-erodible the crest of the evolving mouth bar. beds, (ii) non-aggrading jet flows on erodi- 152 Marine and River Dune Dynamics – MARID VI – 1-3 April 2019 - Bremen, Germany by the initial densimetric Froude number, the sediment-grain size and the sediment supply. The aspect ratio (length vs. width) was controlled by the densimetric Froude number and increased with higher Froude numbers (Fig. 2). The depth of the scour increased with decreasing densimetric Froude number and more gravitationally- dominated flows. The mouth-bar height in- creased with increasing scour depth and was therefore also controlled by the densimetric Froude number. Experiments with aggrading jet flows led to the vertical and lateral Figure 2. Aspect ratio (length/width) of the mouth- growth of the mouth bar and an infilling of bar crest plotted against the initial densimetric Froude the scour (Fig. 1D). The dimensions and number. steepness of the mouth bar and bedforms were further related to the sediment-grain The decelerated density flow was pushed size with coarser grain sizes causing the away by the jet flow, promoting the for- formation of higher and steeper bars and mation of an underflow (Fig. 1B). Under- bedforms. Internally, mouth bars were char- flows were observed to flow all the way to acterized by prograding foreset-like geome- the distal margins of the plate. In experi- tries (Fig. 1D). ments with low-density jet flows, a rise of a Very low-relief rounded bedforms were buoyant plume was observed. However, observed to form on the distal slope of the rising plumes were also observed to develop mouth bar, where the flow had transitioned from high-density jet flow. to a fully gravity-dominated density flow. Successions formed by the experimental The rounded bedforms on the mouth-bar flows comprised early-stage bedforms, front appeared to be in-phase with the densi- scours and mouth bars and bedforms depos- ty flow. On horizontal plates the mouth-bar ited by the distal density flow (Figs. 1C). deposits passed distally into the early-stage Concentric early-stage bedforms commonly bedforms. The movement and growth of preceded the formation of the scour and these bedforms was very slow and they were mouth bar. The formation of these bedforms starved of sediment, receiving only a small was very rapid and occurred within few fraction of sediment that bypassed the mouth minutes after the start of the flow or sedi- bar. At high sediment supply the mouth bar ment feed, respectively. The geometry of the prograded over the early-stage bedforms. bedforms was primarily controlled by the In experiments on inclined plates density densimetric Froude number. The aspect ratio flows were observed to flow all the way to (length vs. width) of the bedforms crests the distal margins of the plate and led to the increased with increasing densimetric formation of small scours and bedforms be- Froude number, displaying a logarithmic- tween the base of the mouth bar and the dis- style trend (Fig. 2). During the experiments tal margin of the plate, which were aligned the early stage bedforms showed an interac- along preferential flow pathways. tion with the flow thickness, indicating that Bedform trains downflow of the mouth they represent small-scale dunes (Fedele et bar were prominent in runs with fine-grained al., 2017). sediment and low sediment supply, where The formation of scours and mouth bars mouth-bar aggradation was low. The proxi- was observed in all experiments with erodi- mal bedforms were symmetrical, while the ble beds, regardless if sediment was fed into distal bedform were asymmetrical with the jet or not. The geometry and dimensions steeper lee sides. In plan-view the bedforms of the scour and mouth bar were controlled displayed straight crests. Superimposed onto 153 Marine and River Dune Dynamics – MARID VI – 1-3 April 2019 - Bremen, Germany these bedform trains smaller-scale asymmet- rich and bedform successions are indicative rical bedforms occurred. Observations dur- of rapidly waning flows, including deposits ing the runs showed an in-phase relation of hydraulic jumps, antidunes and humpback between the bedform trains and the upper dunes (Powell, 1990; Russell & Arnott, interface of the density flow, indicating that 2003; Winsemann et al., 2009; Lang & Win- they represented antidunes (Fedele et al., semann, 2013; Lang et al., 2017b). Farther 2017). downflow, deposits indicate waning flow Bedform fields laterally adjacent to the and deposition by migrating dunes, (climb- mouth bar were prominent when coarse- ing) ripples and suspension fall-out (Powell, grained sediment was supplied at high rates, 1990; Russell & Arnott, 2003; Winsemann leading to high mouth-bar aggradation and et al., 2009).
Details
-
File Typepdf
-
Upload Time-
-
Content LanguagesEnglish
-
Upload UserAnonymous/Not logged-in
-
File Pages6 Page
-
File Size-