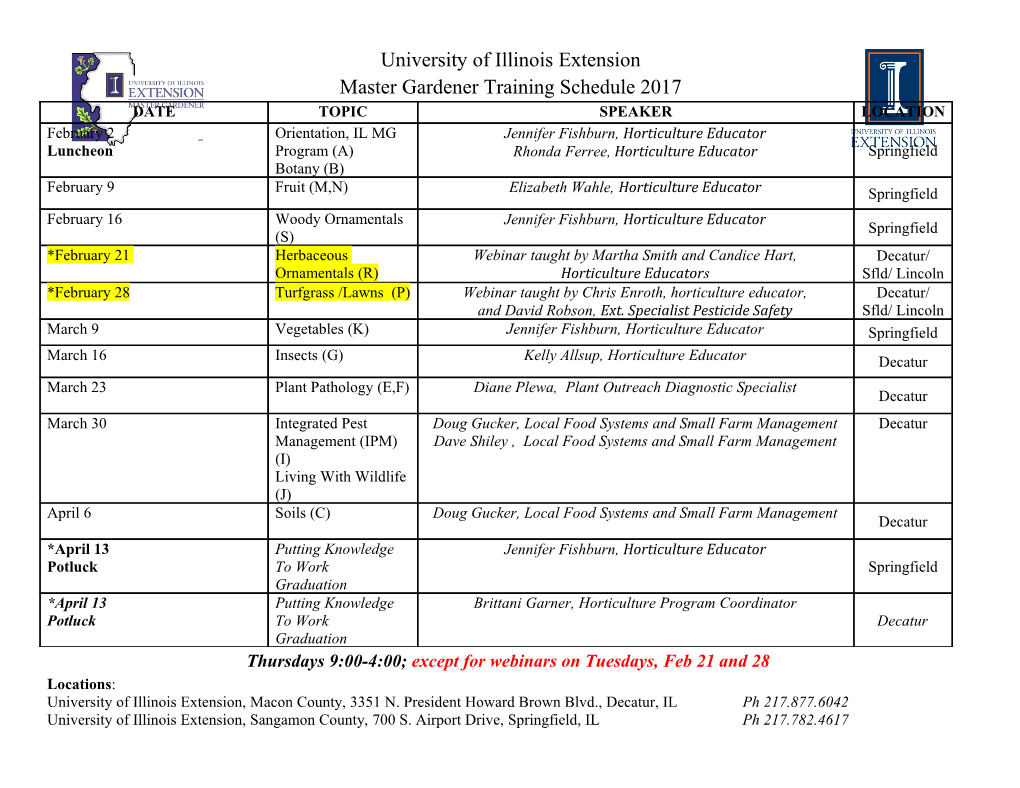
University of Birmingham Ammonia decomposition catalysis using non- stoichiometric lithium imide Makepeace, Joshua W.; Wood, Thomas J.; Hunter, Hazel M. A.; Jones, Martin O.; David, William I. F. DOI: 10.1039/c5sc00205b License: Creative Commons: Attribution-NonCommercial (CC BY-NC) Document Version Publisher's PDF, also known as Version of record Citation for published version (Harvard): Makepeace, JW, Wood, TJ, Hunter, HMA, Jones, MO & David, WIF 2015, 'Ammonia decomposition catalysis using non-stoichiometric lithium imide', Chemical Science, no. 7, pp. 3805-3815. https://doi.org/10.1039/c5sc00205b Link to publication on Research at Birmingham portal General rights Unless a licence is specified above, all rights (including copyright and moral rights) in this document are retained by the authors and/or the copyright holders. The express permission of the copyright holder must be obtained for any use of this material other than for purposes permitted by law. •Users may freely distribute the URL that is used to identify this publication. •Users may download and/or print one copy of the publication from the University of Birmingham research portal for the purpose of private study or non-commercial research. •User may use extracts from the document in line with the concept of ‘fair dealing’ under the Copyright, Designs and Patents Act 1988 (?) •Users may not further distribute the material nor use it for the purposes of commercial gain. Where a licence is displayed above, please note the terms and conditions of the licence govern your use of this document. When citing, please reference the published version. Take down policy While the University of Birmingham exercises care and attention in making items available there are rare occasions when an item has been uploaded in error or has been deemed to be commercially or otherwise sensitive. If you believe that this is the case for this document, please contact [email protected] providing details and we will remove access to the work immediately and investigate. Download date: 27. Sep. 2021 Chemical Science View Article Online EDGE ARTICLE View Journal Ammonia decomposition catalysis using non- stoichiometric lithium imide† Cite this: DOI: 10.1039/c5sc00205b Joshua W. Makepeace,ab Thomas J. Wood,a Hazel M. A. Hunter,a Martin O. Jonesa and William I. F. David*ab We demonstrate that non-stoichiometric lithium imide is a highly active catalyst for the production of high- purity hydrogen from ammonia, with superior ammonia decomposition activity to a number of other catalyst materials. Neutron powder diffraction measurements reveal that the catalyst deviates from pure imide stoichiometry under ammonia flow, with active catalytic behaviour observed across a range of stoichiometry values near the imide. These measurements also show that hydrogen from the ammonia is Received 19th January 2015 exchanged with, and incorporated into, the bulk catalyst material, in a significant departure from existing Accepted 7th May 2015 ammonia decomposition catalysts. The efficacy of the lithium imide–amide system not only represents a DOI: 10.1039/c5sc00205b more promising catalyst system, but also broadens the range of candidates for amide-based ammonia www.rsc.org/chemicalscience decomposition to include those that form imides. Creative Commons Attribution-NonCommercial 3.0 Unported Licence. Introduction ammonia. While some energy must be expended to release the stored hydrogen from ammonia (the thermodynamic minimum Among hydrogen storage candidates, ammonia has one of the energy to completely crack ammonia is 12.7% of the energy À3 highest volumetric (121 kg H2 m at 10 bar) and gravimetric release from the subsequent oxidation reaction), the require- (17.8 wt%) hydrogen densities. At room temperature, it can be ment of excessively high temperatures for the cracking reaction stored as a liquid under moderate pressures (10 bar), which reduces the effective volumetric energy density of the system, allows for conformable, cheap and lightweight plastic tanks and which is particularly detrimental for mobile applications.‡ This article is licensed under a a distribution network similar to that of liqueed petroleum gas Three possible solutions could be employed to mitigate (LPG). These characteristics make it an attractive alternative these issues. Firstly, signicant progress has been made in the fuel and energy buffer.1–3 However, it has been overlooked as a development of ammonia-capture materials (metal halide Open Access Article. Published on 07 May 2015. Downloaded 20/05/2015 13:36:25. viable hydrogen storage system because of real and perceived ammines) on porous supports for the purpose of removing safety issues associated with its use, and the unavailability of an small amounts of ammonia from hydrogen gas streams,5 which effective and inexpensive method of cracking ammonia at could assist in lowering the operating temperature required of moderate temperatures to release its stored hydrogen (eqn (1)): the ammonia decomposition catalyst. These materials have been shown to be capable of delivering <0.1 ppm ammonia = 1 = 3 NH ð Þ4 2 N ð Þ þ 2 H ð Þ: (1) 3 g 2 g 2 g down from an inlet concentration in excess of 10 000 ppm. Secondly, an alternative fuel cell technology such as alkaline A 2006 U.S. Department of Energy report4 concluded that the fuel cells, which have been shown to be unaffected by quite high current ammonia cracking technology was too large and levels (up to 9%) of ammonia in the hydrogen stream,6 could be expensive to be viable for mobile applications. It was also noted used. Although these fuel cells have not been developed as that a high temperature of operation is required to drive the intensively as PEM fuel cells over the last few decades, the ammonia decomposition reaction to completion and thus technology is known to be effective.7 Thirdly, the ammonia may produce hydrogen of very high purity. This purity is required be combusted instead of used in a fuel cell; ammonia alone is because proton exchange membrane (PEM) fuel cells are difficult to ignite, but mixtures of ammonia containing as little irreparably degraded by low part-per-million concentrations of as 10 vol% hydrogen gas have been shown to combust well.8 Regardless of which of these technological solutions is employed, the need to optimise the efficiency of the ammonia aISIS Facility, Rutherford Appleton Laboratory, Harwell Oxford, Didcot, OX11 0QX, UK. E-mail: [email protected] cracking reaction will drive the search for more active catalysts bInorganic Chemistry Laboratory, University of Oxford, Oxford, OX1 3QR, UK for the decomposition reaction, forming a central hurdle to the 4 † Electronic supplementary information (ESI) available: Data analysis procedures, development of ammonia as an alternative to fossil fuels. The ff data tting procedures, X-ray di raction pattern of Li2ND, details of reduction of state-of-the-art ammonia cracking technology for the produc- LiND2 unit cell, POLARIS blank cell results, NPD patterns, exponential ts to QGA tion of high purity hydrogen streams at high ow rates is based data and long-duration reaction data. See DOI: 10.1039/c5sc00205b This journal is © The Royal Society of Chemistry 2015 Chem. Sci. View Article Online Chemical Science Edge Article on ruthenium, oen with complex support architecture and exiting the reactor were characterised by Quantitative Gas promoter species.9,10 Ruthenium is a rare and expensive metal, Analysis (QGA) using a Hiden Analytical HPR-20 QIC R&D mass and so the search for replacement catalysts is an important part spectrometer system. The mass-to-charge (m/z) values routinely ff of the e ort to make ammonia cracking feasible for transport monitored in these experiments were 2 (H2), 17 (NH3), 28 (N2), and stationary applications. 32 (O2) and 40 (Ar). The gas ow rates in to and out of the We have recently reported a method for the decomposition reactor, the reactor temperature and system pressure were also of ammonia, showing similar performance to a ruthenium- recorded. based system,11 that is effected by the concurrent formation and Variable temperature ammonia decomposition experiments decomposition of sodium amide. The generalised mechanism were performed by loading 0.5 g of the catalyst powder into the drawn from the sodium-based system facilitates the decompo- reactor in an argon-lled glove box. Sodium amide (95%, Sigma sition of ammonia through the decomposition and formation of Aldrich) and lithium amide (hydrogen storage grade, Sigma the metal amide (MNH2) from the metal (M), Aldrich) were used as received. Samples of silica/alumina-sup- Æ 1 = ported nickel (66 5% nickel, Alfa Aesar) or alumina-supported MNH2ðlÞ/MðlÞ þ 2 N2ðgÞ þ H2ðgÞ; (2) ruthenium (5% ruthenium, Alfa Aesar) were lightly ground to 1 = remove any agglomeration and were reduced under owing MðlÞ þ NH3ðgÞ/MNH2ðlÞ þ 2 H2ðgÞ: (3) ammonia for 5 hours at 680 C prior to the decomposition experiment. Once loaded with the sample, the reactor was Other metal amides known to thermally decompose via this sealed, transferred to a vertical tube furnace (Severn Thermal route (such as potassium and rubidium amides12) should Solutions) and connected to the gas control panel. The panel display ammonia decomposition activity through a similar was rst ushed with argon and then evacuated up to the mechanism. Conversely, amides which form the corresponding reactor, before ushing the panel and reactor with ammonia imide upon heating are not expected to follow the stoichio- prior to heating. ffi Creative Commons Attribution-NonCommercial 3.0 Unported Licence. metric ammonia decomposition indicated in eqn (2). In this The ammonia conversion e ciency of each catalyst (along paper, we test the ammonia decomposition activity of lithium with that of the empty reactor as a baseline) was measured amide, an imide-forming metal amide. under an ammonia ow of 60 standard cubic centimetres per – Lithium amide (LiNH2), a compound widely investigated for minute (sccm) in the temperature range of 250 650 C.
Details
-
File Typepdf
-
Upload Time-
-
Content LanguagesEnglish
-
Upload UserAnonymous/Not logged-in
-
File Pages12 Page
-
File Size-