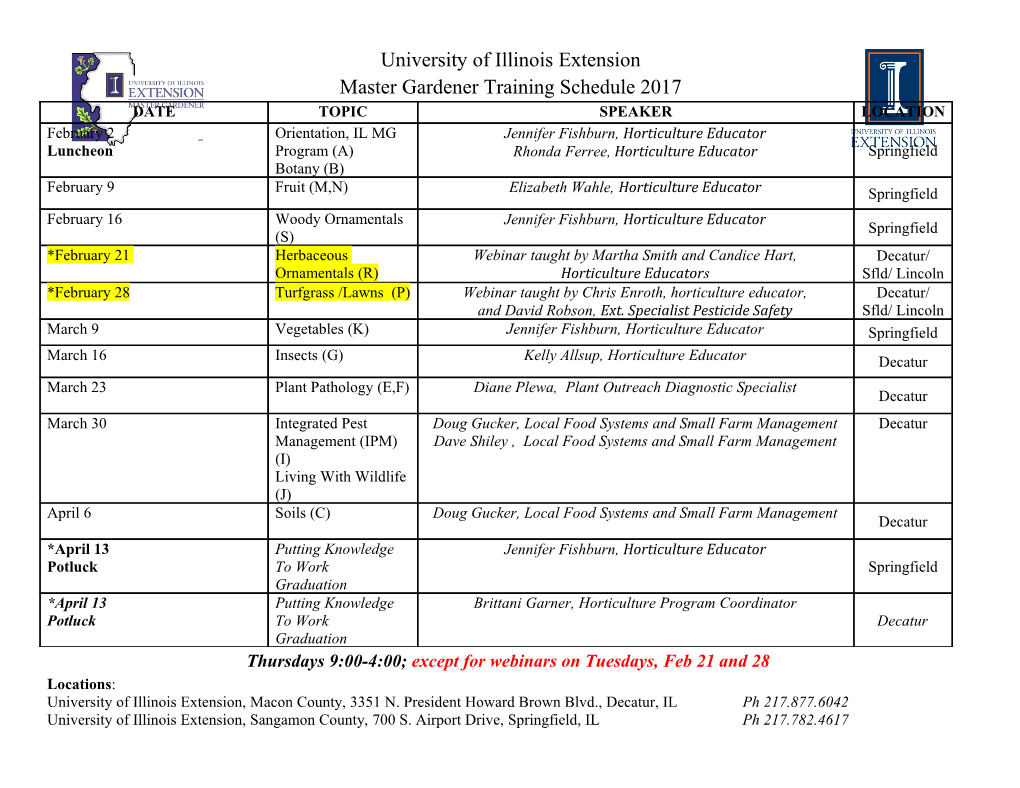
How do Stemmata Grow? The Pursuit of Emmetropia in the Face of Stepwise Growth A thesis submitted to the Graduate School of the University of Cincinnati (Division of Research and Advanced Studies of the University of Cincinnati) In partial fulfillment of the requirements for the degree of Master of Science In the Department of Biological Sciences of the College of Arts and Sciences 2014 By Shannon Werner B.A. Theater, Northern Kentucky University (2005) B.S. Neuroscience, University of Cincinnati (2011) Committee Chair: Dr Elke K. Buschbeck, Ph.D. i Abstract However complex or atypical the visual system, all of its components the lens, eye size and shape, retina and nervous system - must be well tuned to one another. As organisms grow, their eyes must be able to achieve and maintain emmetropia, a state where there is a good fit between the optical and receptor portions of the eye. Based on vertebrate studies, emmetropia is accomplished through a combination of initial regulation by genes, followed by homeostatic visual input from the environment for fine-tuning. How quickly and effectively emmetropia can be achieved will influence the ability of an animal to execute visually guided behavior. While there has been ample research into how vertebrates manage eye growth toward emmetropia, this has never been addressed in arthropods, which develop in a stepwise fashion through ecdysis. Of particular interest are larval holometabolous arthropods with camera type eyes, called stemmata in larval arthropods. Stemmata, such as those of the larval eyes of the Sunburst Diving Beetle (Thermonectus marmoratus, Coleoptera, Dytiscidae) must recover emmetropia after ecdysis, developing a well formed lens and receptor components that are well tuned to the lens’ optical properties. Here I use this system as a model for measuring when and where eye growth toward emmetropia occurs around different molting periods. Histological and optical techniques were used to investigate growth of the principal larval eyes of T. marmoratus. These studies revealed that larval eye tubes grew significantly within the first hour after molt, with the crystalline cone contributing the most to overall growth. Lenses were slower than the eye tubes to reform, regaining the ability to project sharp, distinct images eight hours after molt. Further osmotic experiments using immersion in hypotonic (water) or hypertonic (100% insect Ringer’s) solutions explored water uptake as a potential mechanism of growth. Preliminary data showed significant differences in the change of eye tube length for eye two between individuals exposed to water and ii Ringer’s solution. These results are the first demonstration of how holometabolous arthropod stemmata grow and change after a molt. Specifically notable is that the eye tube and optical components grow very rapidly, within the first hour, before the lens is capable of image formation. Results also show that within the first day after molting, larval eyes already regain functional emmetropia, and that water uptake within the eye via osmosis may be vital to the rapid growth of these larval eyes. I anticipate that this first exploration of how stemmata grow after molting will open new avenues of investigation in other arthropod camera type eyes or investigations into comparisons between post-ecdysal camera type eye development in aquatic arthropods land based land based arthropods. Additionally, preliminary evidence of osmosis as a potential mechanism of growth may provide a basis for a thus far unknown mechanism for invertebrate eye growth. Further investigation into water regulation in vertebrate and invertebrate eyes as a mechanism for growth and fine-tuning are warranted. Keywords: Emmetropia, Eye growth, Arthropod, Vision, Stemmata iii iv Acknowledgements I would like to thank my advisor Elke Buschbeck for her enthusiasm for science and teachinG, her unsurpassable enerGy for any and all thinGs we miGht learn, her willinGness to allow students take on unusual projects with potential for openinG new avenues into exploration of invertebrate eyes. Elke has Gone above and beyond, and shown me compassion in times of stress and injury. I would like to thank my committee members: Dr John Layne, whose Sensory PhysioloGy course kicked my brain into motion, where I found a niche no one else had yet explored for this project. For Dr Tiffany Cook, for her incredibly sharp eye for improvement and all the knowledge she brings, which pushed me to work harder. Thanks to Ron Debry extendinG GAC support and funding for the final semester stretch of my thesis work, with consideration for the major back injury I sustained and the lonG process of recovery. Special thanks to Randy MorGan and the Insectarium of the Cincinnati Zoo & Botanical Garden provided the initial culture of Sunburst divinG beetles, and to Brian HodGe for his willinGness to lend his expertise on all matters divinG beetle. This research was funded by the National Science Foundation under Grants IOS0545978 and IOS1050754, and in part by the University of Cincinnati’s Weiman Wendel Benedict Student Award in 2012 and 2013. Special thanks go out to my friends and Prem Rajkumar, Sri Pratima Nandamuri, Jessie Ebie, Srdjan Maksimovic, Aaron Stahl and Jeremy Didion, and Luke HonG. HavinG these wonderful, and intelligent people in my life really helped me throuGh Grad school, happy to talk science and brainstorm, or just relax and enjoy the sort of life it is hard to explain to people who are not in your field. They made me feel as thouGh I belonGed somewhere, my “science family.” I am eternally indebted to my father Richard and stepmother Mary for their unconditional love and support. Of all my relatives I owe my sister, AshleiGh, the most. We were the closest of friends and sisters growing up as Marine Corps Brats, and carried that into adulthood. Then there is Matthew, my beacon of sanity and calm, who listens and provides a safe place to fall when I stumble and who is the best co-caretaker I could ask for when it comes to our wonderful, weirdo doGs, Ari and Tina. v Table of Contents Introduction – 1 Materials and Methods – 7 Initial behavioral experiments - 7 EstablishinG an appropriate timeline – 7 Animal rearinG and surveillance – 8 SamplinG for histoloGy and optics – 9 Histology processinG and staininG – 9 HistoloGical imaGinG and measurements - 10 Optical imaGinG and imaGe contrast measurement – 11 HistoloGical and optical comparisons and statistical analysis - 12 Point of molt osmotic comparisons – 13 Results - 15 Histological observations – 15 Optical observations – 19 Optical and retina fit – 21 Post molt osmotic observations – 22 Discussion - 23 ChanGes in eye size - 23 Lens reformation and fit of imaGes with retinas – 28 Preliminary evidence for an osmotic method of Growth – 30 Summary - 32 Figures – 34-45 Works Cited – 46-49 vi List of Figures and Tables Figure 1) A- An electron microscopy image of the whole larval head. B- Schematic of a sagittal section of the larval eyes showing the positions of the primary eyes 1 and 2 and their retinal layout. C- Diagram of the life cycle of T. marmoratus, from egg to adulthood, all phases are shown to scale. D, - sagittal section of a first instar with back of the lens to end of eye measurements for eyes 1 and 2, D’- sagittal section of a second instar with measurements for eye 1 and 2 , D’’- sagittal section of a third instar with measurements for eyes 1 and 2. Pp - 34 Table 1) For an example second instar molt time of 9 AM, this outlines sampling times, their time points, and notations for each time point. Pp - 35 Figure 2) Chart of third instars average total number of larvae captured by day. Pp - 35 Figure 3) Histological measurements taken for each eye. A- Back surface of the lens to start of distal retina, B- Back surface of the lens to the distal retina pit, C- Back surface of the lens to the end of the distal retina/start of the proximal retina, D- Start of the proximal retina to the end of the proximal retina, E- Back surface of the lens to the end of the eye tube. Pp - 36 Figure 4) Scatter plot of mean values for major Eye 1 histology points. Symbols correspond to the major landmarks that are shown on the diagram of the eye. Diamond shows the end of the crystalline cone and start of the distal retina, squares show the end of the distal retina and the start of the proximal retina, triangle shows the end of the proximal retina, and circles indicates the end of the entire eye capsule. Pp - 37 Figure 5) Scatter plot of mean values for major Eye 2 histology points. Symbols correspond to the major landmarks that are shown on the diagram of the eye. Diamond shows the end of the crystalline cone and start of the distal retina, squares show the end of the distal retina and the start of the proximal retina, triangle shows the end of the proximal retina, and circles indicates the end of the entire eye capsule. Pp - 38 Figure 6) Mean values for the length of the crystalline cone regions. A. Eye 1. B,Eye 2,. Significant differences between time points are marked with *. Pp - 38 Figure 7) Mean values for the total retinal area (distal retinal length and proximal retina length). A. Eye 1. B,Eye 2,. Significant differences between time points are marked with *. Pp - 39 Figure 8) Diagram showing a comparison of average major landmark sizes for eye 1 of final day of Second Instar (day 5), and first day of third Instar (at twenty hours). It also shows growth values for each portion of the eye (between these time points), and the percent of the total growth of the eye that each area represents.
Details
-
File Typepdf
-
Upload Time-
-
Content LanguagesEnglish
-
Upload UserAnonymous/Not logged-in
-
File Pages58 Page
-
File Size-