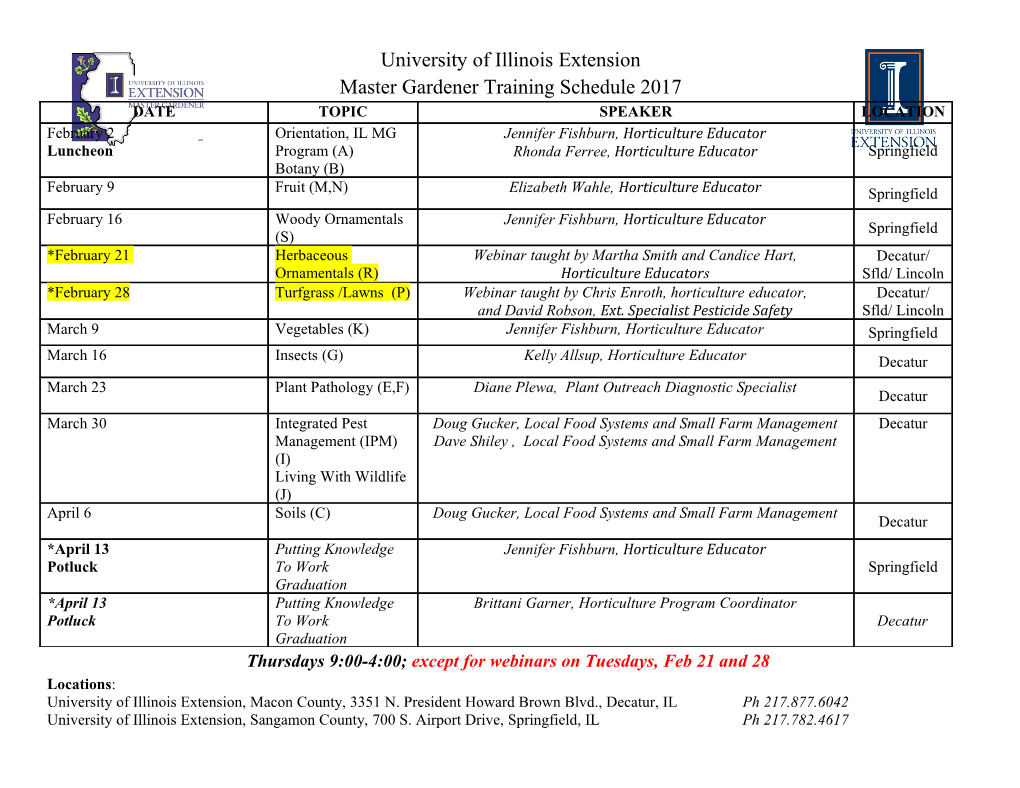
PROTONATION AND OTHER ACID-BASE CHEMISTRY OF CHLOROPHOSPHAZENES A Thesis Presented to The Graduate Faculty of the University of Akron In Partial Fulfillment of the Requirements For Degree Master of Chemistry Bang Hoang August, 2005 PROTONATION AND OTHER ACID-BASE CHEMISTRY OF CHLOROPHOSPHAZENES Bang Hoang Thesis Approved: Accepted _______________________________ _____________________________ Advisor Dean of the College Dr. Claire Tessier Dr. Charles Monroe _______________________________ _____________________________ Faculty Reader Dean of the Graduate School Dr. Wiley Youngs Dr. George Newkome _______________________________ _____________________________ Department Chair Date Dr. David Perry ii DEDICATION This is dedicated to my parents, Be and Cam Hoang, for their tireless, hard work and sacrifice that made everything possible. And to Audry for her strength and faith. iii ACKNOWLEDGEMENTS A very special thanks goes Dr. Claire Tessier for her guidance, knowledge and friendship. Her sincerity and wisdom will never be forgotten. Also a heartfelt thanks must go to Amy Heston and Tatianna Eliseeva for the countless times they have aided me in the lab. Thank you to Dr. Wiley Youngs and his group for their help through the years. Finally, thanks to Alyison Leigh for her assistance with mass spectra. iv TABLE OF CONTENTS page Table of Figures ……………………………………………………………………….. vi CHAPTER I. INTRODUCTION …………………………………………………………….. 1 II. EXPERIMENTAL ...…………………………………………………………... 11 III. RESULTS AND DISCUSSION ...………...………………………………….. 15 IV. CONCLUSION ...……………………………………………………………... 29 REFERENCES ...…………...…..…………………………………………………….... 31 v LIST OF FIGURES Figure page 1. Phosphazene Trimer and Linear Phosphazene …………………………..… 1 2. Allcock Mechanism. ……………………………………..…………………….. 4 3. Sulkowski Mechanism3 …………………………………….…………………. 6 31 4. P NMR Spectrum of [PCl2N]n Obtained n the Synthesis of (PCl2N)3 Magnified …...………………………....…………….. 16 313 5. P NMR Spectrum of the [PCl2N]n Obtained n the Synthesis of (PCl2N)3 …………………………………..………..…………… 17 6. Mass Spectrum of [PCl2N]n in DCTB ……………………………..………... 19 7. Mass Spectrum of [PCl2N]n in DCTB with NaTFA …………………...…... 20 8. Mass Spectrum of [PCl2N]n in DCTB with LiTFA ………………………… 21 9 1H NMR Spectrum of Magic Acid in Benzene ……………………..……… 22 1 10. H NMR Spectrum of (PCl2N)3 and Magic Acid …………………..........… 23 31 11. P NMR Spectrum of [PCl2N]3 and Magic Acid ………………………..… 24 31 12. P NMR Spectrum of PCl2N]3 and Magic Acid Magnified …...…............. 24 1 13. H NMR Spectrum of Commercial [PCl2N]n with HOTf ………...…......... 25 31 14. P Spectrum of [PNCl2]n and HOTf …….…..………………….…………... 27 vi CHAPTER 1 INTRODUCTION The term “phosphazene” is used to encompass a class of compounds that contain one or more subunits of “-P=N-” in their structures. These compounds can vary greatly in size from several atoms to large polymers. In the polymers, nitrogen and phosphorus atoms alternate to make up the backbone with single and double bonds between them also alternating. The examples given below (Figure 1) are a phosphazene trimer, (NPR2)3, and linear polyphosphazene, [NPCl2]n. On the phosphorus atom, there are two substituents often represented by symbol “R”. R can be an amine, alcohol, akyl or aryl groups or a halogen in the inorganic polymer. RR P R N N RR PN P P R N R R n Figure 1. Phosphazene Trimer and Linear Phosphazene 1 In the 1890's, H. N. Stokes was studying compounds that formed when phosphorus pentachloride reacted with ammonia.1 He was the first to put forth the possible structure (NPCl2)3 and also other homologues. Further he accounted how, when heated, these compounds would form an “inorganic rubber.” In a time before even the concept of polymers was commonly accepted, Stokes had suggested that this rubber was in fact a polymer. Four decades later in 1936, X- ray diffraction studies performed by Meyer, Lotmar and Pankow confirmed Stokes' belief. In 1948, Goldschmidt and Dishon attempted to use organic molecules to replace the chlorine atoms in the rubber but were unsuccessful. The area of phosphazene research sat largely dormant until the mid 1960's when Allcock, Kugul and Valan published a series of papers on the subject. They described how the inorganic rubber's P-Cl bond was hydrolytically unstable. The unstable inorganic polymer could be used as a precursor for a number of interesting substituted polymers. Additionally they showed that the [PCl2N]n polymer could be dissolved in a number of solvents such as benzene, toluene, cyclohexane and tetrahydrofuran. Insoluble rubbers, they said, were crosslinked. These papers inspired great interest and research. That curiosity still lives. Phosphazenes hold great promise as a class of compounds due to their very interesting properties and potential uses. Linear polymers have great flexibility over a wide range of temperatures and are chemically stable to acid, bases and other reactive chemicals.1 These traits make them ideal for uses such 2 as O-rings and gaskets. The polymer is also heat resistant and flame retardant and could be used in fire-resistant materials such as textiles. Cyclic oligomers 2 have been suggested for use in antitumor drugs, insect control and fertilizer. Polydichlorophosphazene [PCl2N]n is the precursor for many phosphazene compounds. The most common synthesis involves the melt 1 polymerization of (PCl2N)3. This ring opening polymerization (ROP) is performed by heating the trimer to 250° C for about 8 hours. Longer reaction time and higher temperature can lead to cross-linking the polymer. The crosslinked polymer is generally considered an undesirable side product. It has been show that the reaction is catalyzed the presence of a Lewis acid such as BCl3 or AlCl3. Also it appears that water in trace amounts is needed to catalyze the reaction.3 This is evidenced by the readily proceeding polymerization in glass reaction flasks but not in dried quartz phials. Glass walls are known to hold onto water in minute amounts even when thoroughly dried. However in quartz vials, water can be driven off much more thoroughly. The inability to polymerize in quartz is believed to be caused by this lack of trace moisture. Although the ring opening polymerization has been long known, the mechanism by which the polymerization occurs is still up to speculation. One mechanism is suggested by Allcock and Best (Figure 2).1 Noting that the presence of a Lewis acid promotes ROP, they propose that a Cl- anion first disassociates from the trimer. This leaves phosphorus with a positive formal 3 Cl- Cl Cl Cl Cl Cl P P P N N N N Cl Cl N N Cl Cl Cl Cl P P P P P P N N Cl N Cl Cl Cl Cl Cl Cl Cl Cl Cl P N PNP Cl Cl N Cl Cl NP P N N Cl Cl Cl n P P N Cl Cl Figure 2. Allcock Mechanism1 charge. The phosphorus can then be attacked by a nitrogen atom from another ring. The attack forms a bond between the phosphorus and attacking nitrogen. Also it opens the ring of the attacking nitrogen. The phosphorus atom that used to be next to the attacking nitrogen is then left with a positive charge. This phosphorus atom serves as a site available for the continuation of the polymerization. Disassociation of the Cl- ion in the melt is supported by conductivity studies and the catalyzing nature of the presence of Lewis acids. Further, polymerization is inhibited by the substitution of chlorine with organic groups that are less likely to disassociate. An intriguing examination of the initial stages of polymerization based on mass spectrometric studies was reported by Traldi.4 This report is quite novel because mass spectroscopy is usually used to obtain structural data. 4 Traditionally, examination of the mass to charge ratios (m/z) of mass spectra leads to the masses of the compound and its fragments. By analysis of the masses of the fragments, structural data about the analyte can be gained. For instance, the mass spectra of a polymer will often contain a series of regularly- repeating m/z’s. The difference between these peaks represents the mass of the repeat unit of the polymer. In this particular report,4 Traldi noted that when the mass spectra of (PCl2N)3 (m.w. 345) was obtained using electron ionization techniques (EI), the m/z of 310 was prominent, a difference of 35 from the trimers molecular weight. This corresponds to the loss of Cl-. Recognizing this as the initial step of the Allcock’s proposed mechanism, Traldi attempted to induce polymerization inside the ionization chamber. He switched from EI to chemical ionization (CI). This had the effect of raising the pressure inside the chamber to 1 torr. He also increased the temperature of the chamber to 200°C. When the mass spectra were then obtained, m/z’s corresponding to the masses of [PCl2N]n with 4 ≤ n ≤ 21 were observed. Demonstration that ionization causes the loss of Cl- from the trimer and then that polymerization can occur in the ionization chamber lends credence to Allcock’s mechanism. Sulkowski suggests an alternative mechanism to Allcock’s.3 In Sulkowski’s mechanism (Figure 3), a nitrogen atom on the chain is protonated to form a linear azophosphonium cation. He suggests that the proton source could be from trace amounts of water left on the walls of the reaction vessel. The water 5 Cl Cl Cl Cl Cl Cl P P H P N N NH H+ Cl Cl Cl P P P N Cl N Cl N Cl Cl Cl N p NP N3P3Cl6 P Cl N N - HCl Cl Cl P P N Cl Cl Figure 3. Sulkowski Mechanism3 hydrolyzes the P-Cl bonds to give small amounts of HCl. This protonation could lead the P-N bond scission and make phosphorus available for nucleophilic attack. The nitrogen from a trimer could attack and create a larger phosphonium cation. One interesting aspect of Sulkowski’s proposed mechanism is the protonation of the nitrogen on the trimer.
Details
-
File Typepdf
-
Upload Time-
-
Content LanguagesEnglish
-
Upload UserAnonymous/Not logged-in
-
File Pages37 Page
-
File Size-